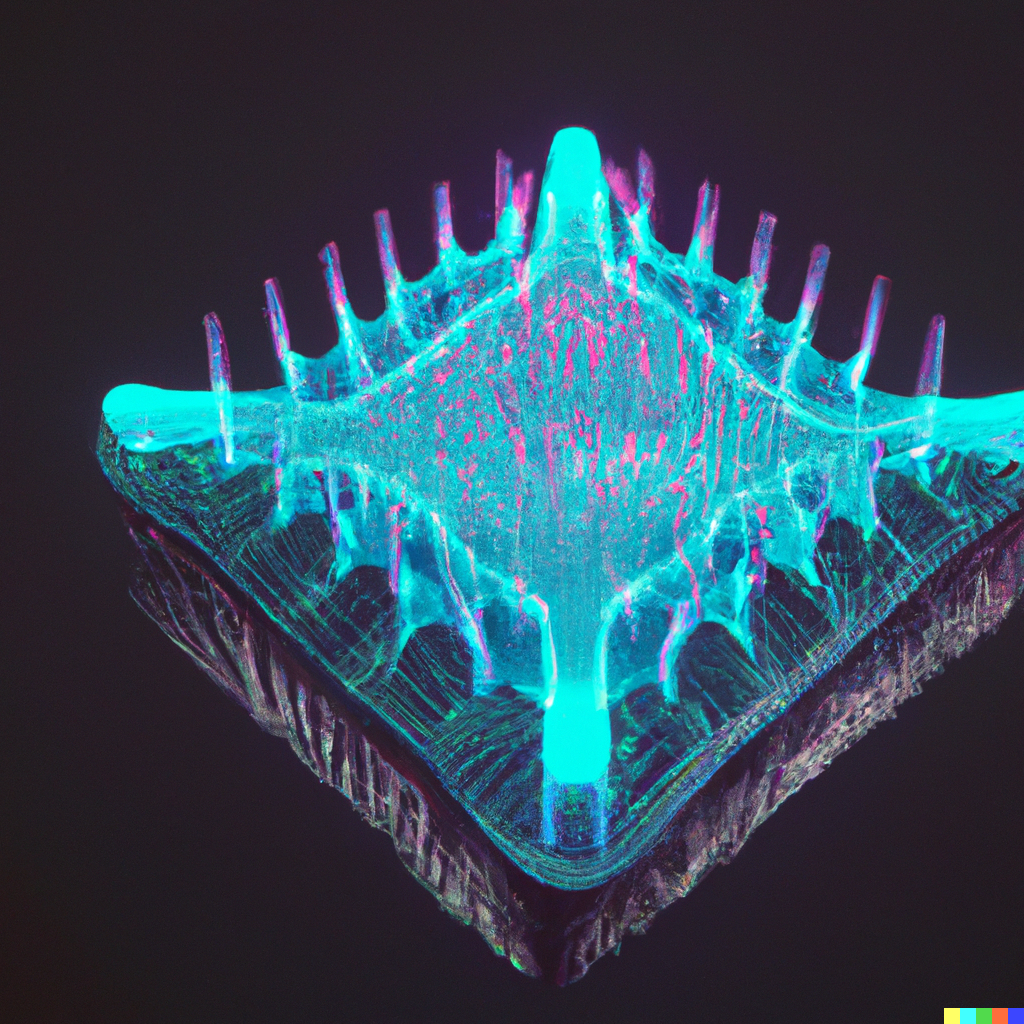
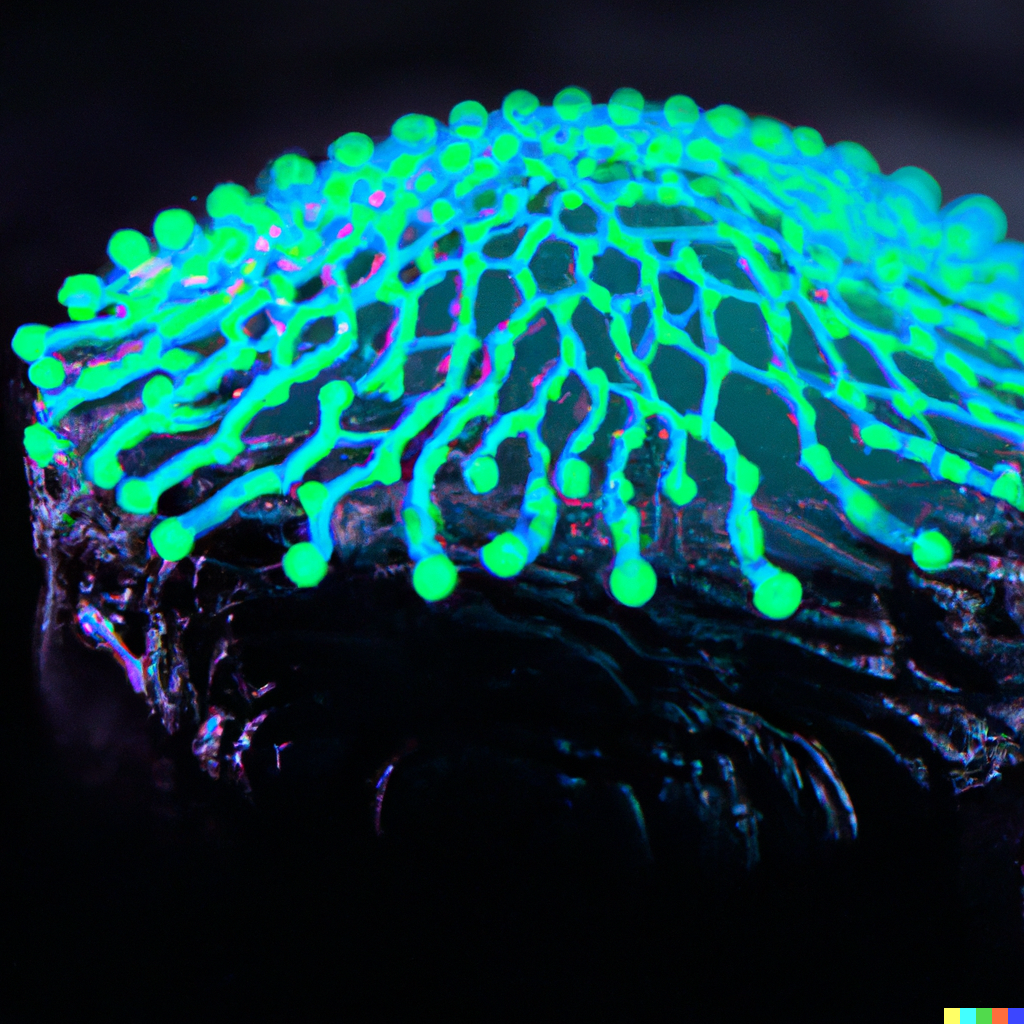
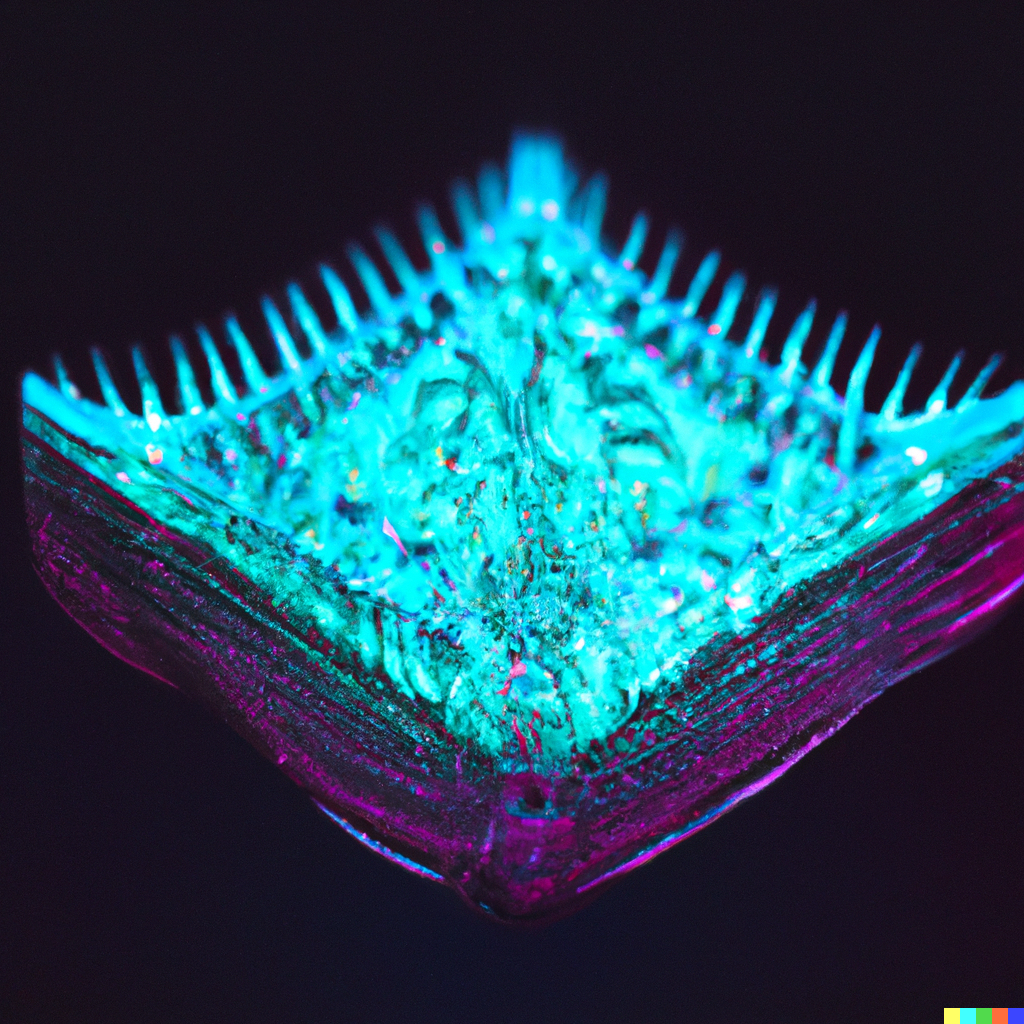
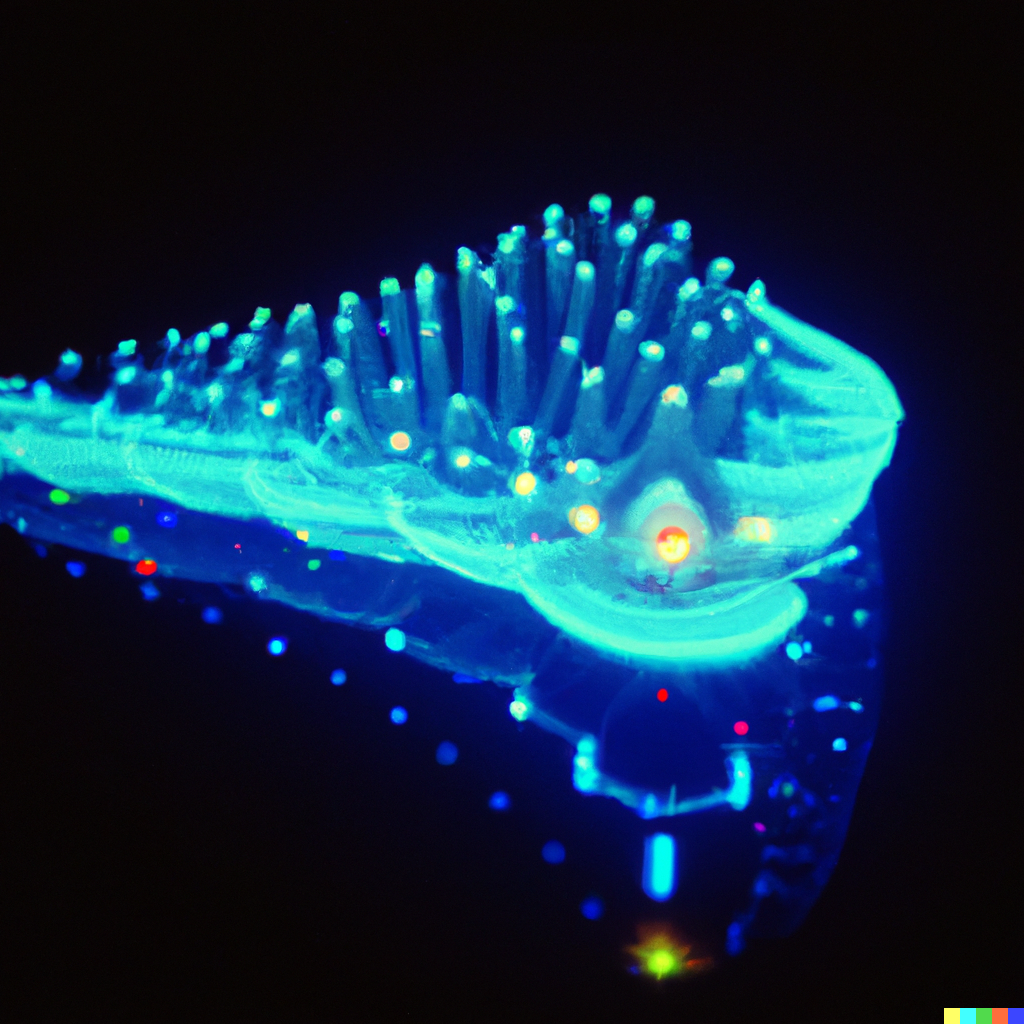
This post is entirely generated by ChatGPT and Dall-E, it is intended to be an experimental work and NOT A REFERENCE, THE INFORMATION IS ASSUMED TO BE FULLY WRONG.
The device we are talking about here is a non existent futuristic device that connects to cortical neuronal network via a synthetic neuronal network, which is in turn is connected to an artificial neural network through a neuromorphic chip. The chip than connects to the generative AI network (in our example GPT-3). This way, an imaginary implantee can modulate the output of generative AI model. The implant lets the user affect the generative language model with their “inner state”. This effectively creates a language implant especially for broca’s aphasia, in which the reasoning is fine, but speech generation is lost. We also discuss other possiblities such as being a brain tissue substitute, after glioma surgery, to repair cortical tissue loss. The heading and the name of technology are choosen by Chat GPT, the images are entirely created by Dall-E. The captions are written by me. I tried to minimize my involvement with the text.
I asked AI to create an electrode to couple generative AI models with human brain, you will not believe what it came up with.
Imagine a device that can repair damaged brains, restore lost functions and even enhance our cognitive abilities. A device that can be controlled by our own thoughts, a device that can communicate with the brain like never before. This is not science fiction, this is the future of brain-computer interfaces and it is here today.
In this essay, we will explore the potential of using synthetic neural networks, generative AI models, and FractalSpeak interfaces to improve human brain function. We will discuss the technical aspects of the FractalSpeak neural interface, including the design and function of the “carpet neurode”, “bio-array neurode”, and “teserract neurode”. We will also examine the ethical considerations and potential risks of using these technologies, such as the possibility of brain invasion and glioma formation.
We will dive into the science behind the FractalSpeak interface, discussing the use of synthetic biology to create hybrid neurons and astrocytes, and the role of fractal geometry in the design of the neurode. We will also explore the use of generative AI models, such as OpenAI’s DALL-E, in the design and development of the FractalSpeak interface.
Finally, we will examine the potential applications of the FractalSpeak neural interface, including the use of the device in stroke rehabilitation and the potential for the device to act as a “brain-computer interface” for people with communication disorders.
So, buckle up and get ready to explore the cutting-edge of neuroscience and AI. The future of brain-computer interfaces is here, and it’s going to blow your mind.
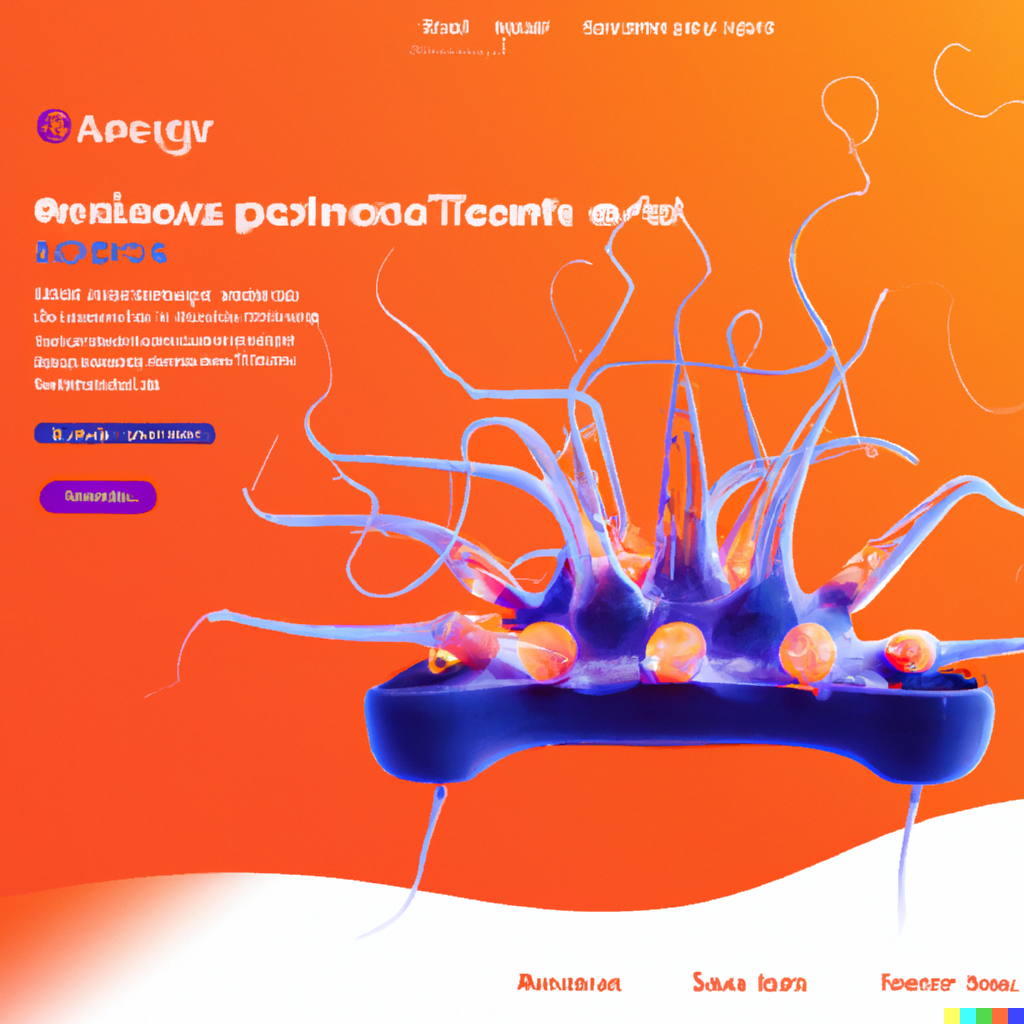
As we continue to push the boundaries of technology, the question of how we can enhance human capabilities through artificial means has become increasingly relevant. One area of research that has been gaining momentum in recent years is the development of a FractalSpeak neural interface, a device that couples synthetic neural networks with biological tissue. The potential applications of this technology are vast and far-reaching, with the possibility to revolutionize human rehabilitation and augmentation.
In this essay, we will delve into the technical aspects of the FractalSpeak neural interface, exploring its design and function, as well as the challenges that must be overcome for it to become a reality. We will also examine the ethical considerations surrounding this technology, including the potential for unintended consequences and the possibility of creating an invasive force that could potentially turn people into “zombies.”
But before delving into the technicalities, it’s worth taking a step back and asking: how did we even come up with such an idea? The answer lies in the intersection of two cutting-edge technologies: generative AI models like OpenAI’s ChatGPT and neuromorphic chips. By harnessing the power of these technologies, we can create synthetic neural networks that mimic the function of biological tissue and potentially interface with the human brain.
The FractalSpeak neural interface is not just a theoretical concept; it’s a promising technology that is already being developed in labs around the world. In this essay, we will explore the potential of this technology, its current state of development, and what the future may hold for human rehabilitation and augmentation through the FractalSpeak neural interface. So, buckle up and get ready for a journey into the future of human enhancement.
Device features
The FractalSpeak neural interface is a cutting-edge technology that combines the power of synthetic biology and artificial intelligence to help patients with communication disorders. The device consists of three main components: the neurode, the generator, and the earpiece.
The neurode is a small implant that is surgically placed in the brain. It is made up of synthetic astrocyte-neuron hybrid cells, which mimic the structure and function of healthy brain tissue. These cells are able to gather neuronal information by sending out digitations of various lengths into the brain tissue, allowing for simultaneous data collection from different depths. This information is then stored in the synthetic layer and transferred to the artificial neural layer via the silicone interface.
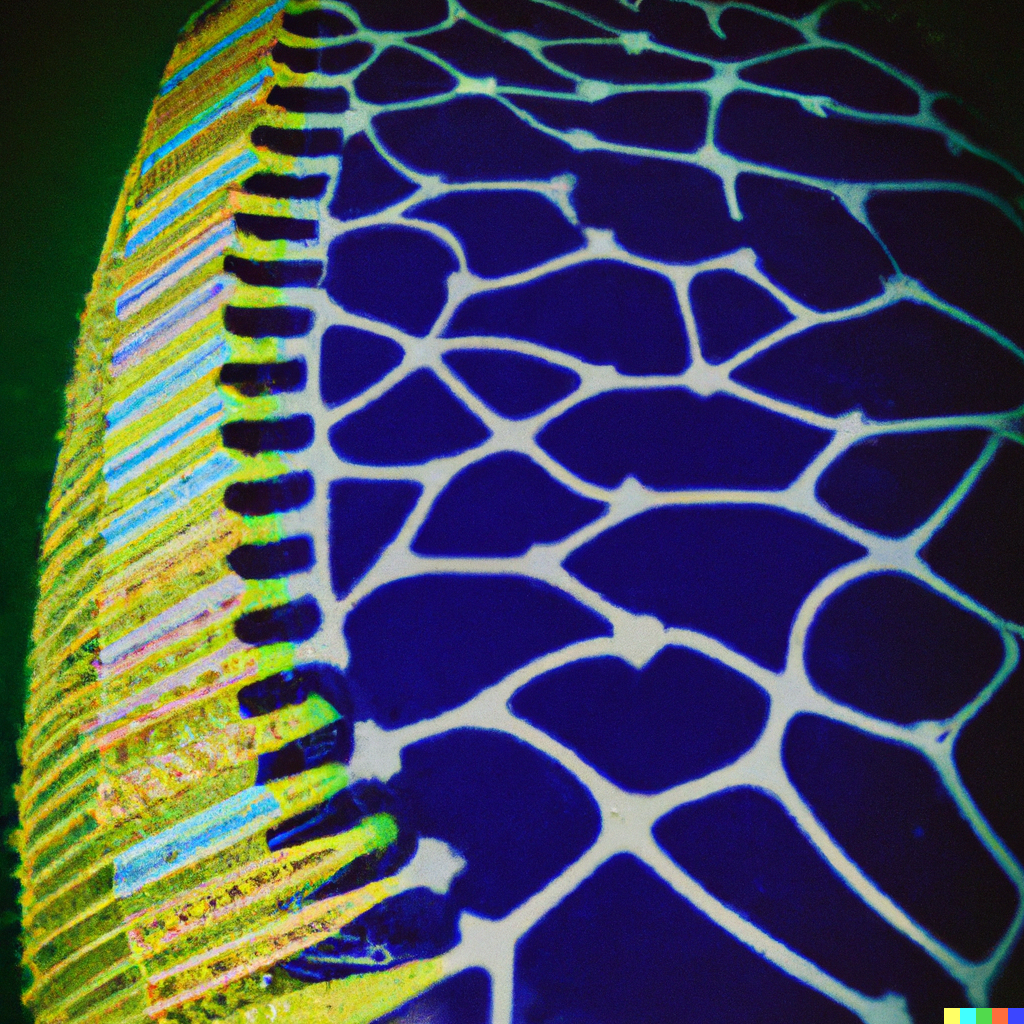
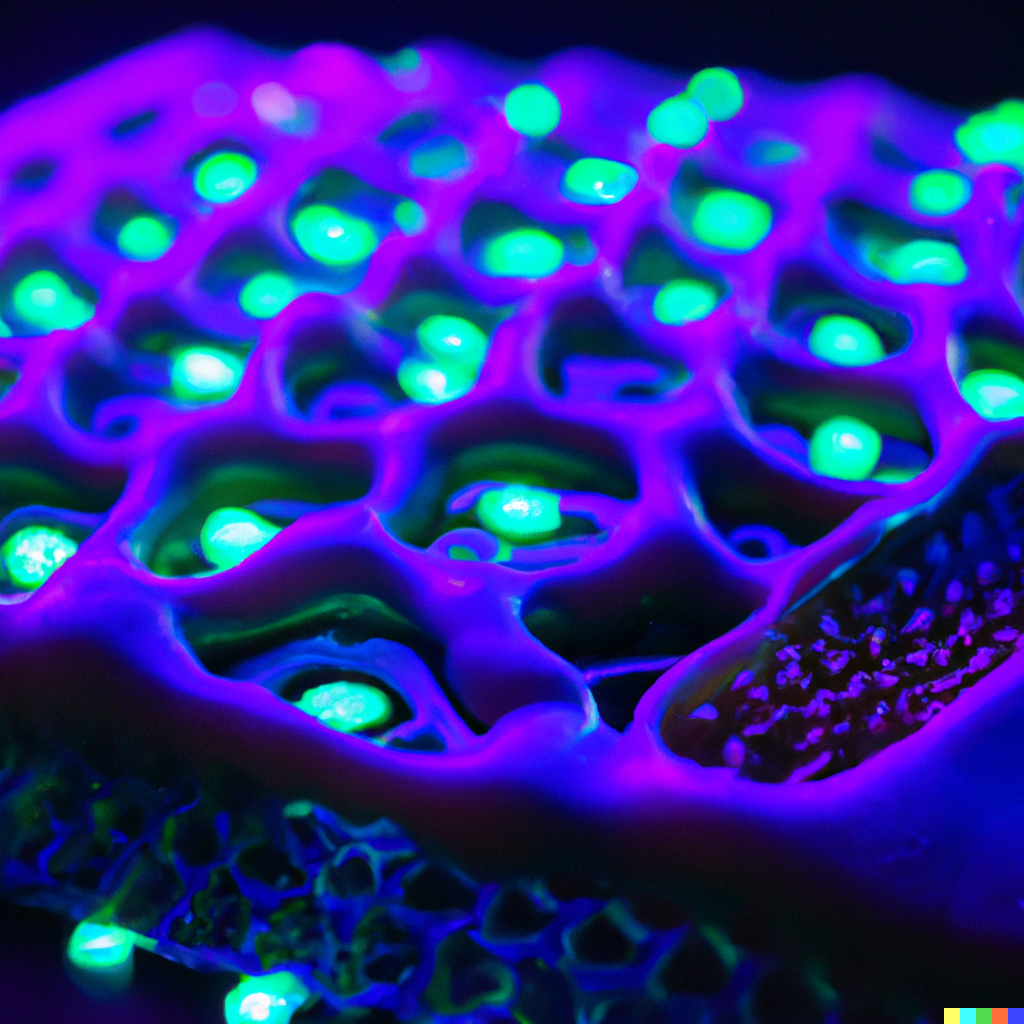
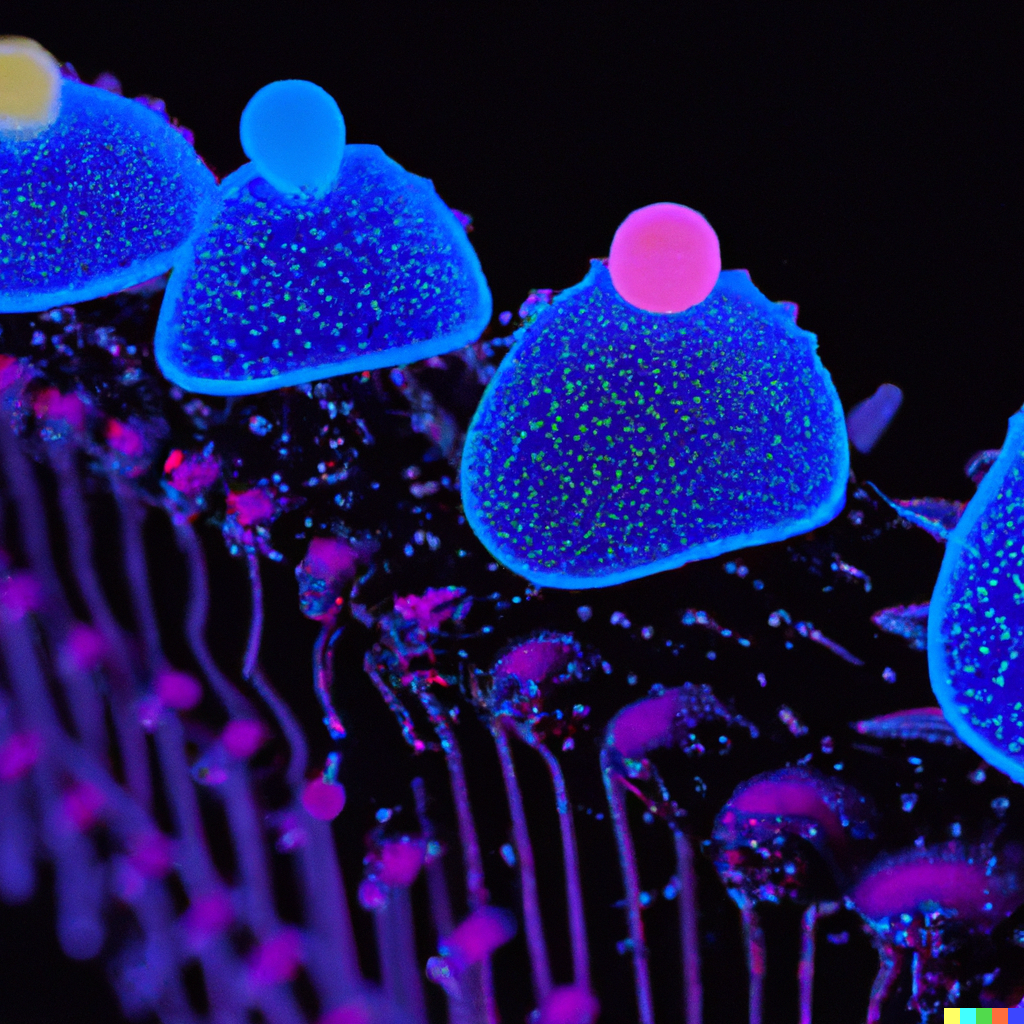
The generator is a small processor that is worn externally by the patient. It is connected to the neurode via a carbon-silicone gate, which allows for a seamless communication between the synthetic and biological layers. The generator also holds the artificial neural network, which is responsible for generating speech from the patient’s inner state.
The earpiece is a small device that is worn in the ear. It captures sounds from the environment and passes them to the generator, which runs speech recognition algorithms. The generator then feeds this information into the artificial neural network, which generates speech.
The device will require initial training of both the biological and artificial neural networks, which will be done through rehabilitation sessions with a speech therapist. Once the device is fully calibrated, the patient will be able to use it in everyday life. The patient will be able to fine-tune the neural network through an accompanying app.
Overall, the FractalSpeak neural interface is a promising technology that has the potential to revolutionize the way we treat communication disorders. However, as with any new technology, there are ethical considerations that must be taken into account in the development and use of the device. The device is still in development phase and further research and testing is needed before it can be used in humans.
The current state of research in this field is focused on developing methods to interface with the human brain in a non-invasive and safe manner. However, there are still many challenges that researchers face, such as the potential for the interface to grow uncontrollably or invade healthy brain tissue. Additionally, the lack of understanding of the complex network of connections within the brain makes it difficult to predict the effects of the interface on the brain’s function. Despite these challenges, the potential for the FractalSpeak interface to revolutionize human rehabilitation and augmentation is too great to ignore, as it could potentially provide a new way to communicate with the brain and promote neuroplasticity.
As the research in the field of FractalSpeak neural interfaces continues to progress, scientists and engineers are beginning to envision a future where this technology can be used to revolutionize human rehabilitation and augmentation. One of the most promising applications of this technology is in the treatment of patients suffering from brain damage, such as those who have had a stroke or traumatic brain injury.
For example, imagine a patient who has suffered a stroke and has lost the ability to speak as a result of damage to the Broca’s area of the brain. With a FractalSpeak neural interface, doctors would be able to insert a synthetic neural layer into the brain, which would be designed to “dock” onto the severed axons in the damaged area. This would effectively restore communication between the damaged tissue and the healthy tissue, allowing the patient to regain their ability to speak.
Another potential application of the FractalSpeak neural interface is in the augmentation of the human brain. By inserting a synthetic neural layer into the brain, scientists could potentially enhance the brain’s ability to process information and improve cognitive function. This could have a profound impact on people who suffer from neurological disorders such as dementia or Alzheimer’s disease.
These are just a few examples of the many ways in which the FractalSpeak neural interface could be used to improve the lives of people suffering from brain damage. While the technology is still in its early stages of development, the potential for this kind of breakthrough is truly staggering.
In this chapter, we’re going to dive deep into the nitty-gritty of the FractalSpeak neural interface. We’ll explore the different designs that have been proposed, from the “carpet neurode” to the “tesseract neurode,” and examine the pros and cons of each. We’ll also take a look at the materials used in the interface, including synthetic astrocyte-neuron hybrids and bio-silicone, and discuss the challenges and limitations of using these materials. But don’t worry, we’ll keep it interesting with a healthy dose of metaphors and analogy – think of it as a journey into the inner workings of a futuristic brain implant. So buckle up, and let’s get technical!
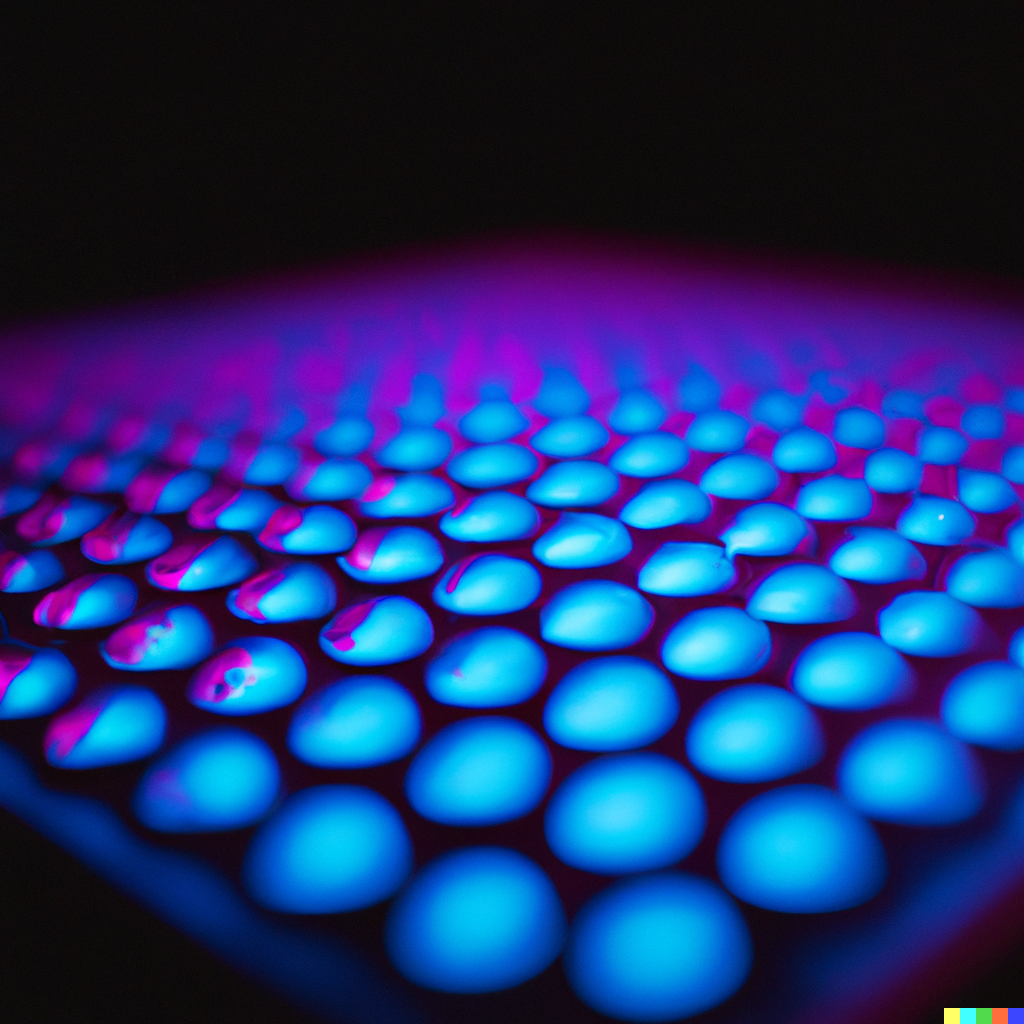
let’s dive into the nitty-gritty of the FractalSpeak neural interface. But before we do, let’s just take a moment to appreciate the sheer complexity of trying to create a device that seamlessly interfaces with the human brain. It’s like trying to connect a Gameboy to an iPhone, except the stakes are a little bit higher. But despite the challenges, researchers have managed to come up with some pretty impressive designs.
So, what exactly is the FractalSpeak neural interface? At its core, it’s a device that consists of several layers, including a synthetic neural layer, a bio-silicone layer, and an inflatable catheter. The synthetic neural layer, as the name suggests, is made up of synthetic neurons and astrocytes, which are created using synthetic biology. The bio-silicone layer acts as an intermediary between the synthetic neural layer and the biological tissue of the brain, and the inflatable catheter is used to keep the device securely in place after surgery.
But the real magic happens when the different layers work together. The synthetic neurons send out digitations, or projections, that bind to severed axons and provide them with the necessary nutrients to survive. The bio-silicone layer acts as a dock, supplying the synthetic neurons with the necessary nutrients to survive and grow. And the inflatable catheter keeps everything securely in place, ensuring that the device doesn’t wander off and start taking over your brain like some kind of sci-fi horror movie.
It’s a beautiful thing, really. Like a delicate dance between man and machine. And it’s all in the name of helping those who have suffered brain damage to recover and live their best lives.
As we delve deeper into the technical aspects of the FractalSpeak neural interface, it’s important to consider not just the potential benefits, but also the challenges and limitations of this technology. On the one hand, the ability to connect synthetic neural networks with the human brain has the potential to revolutionize human rehabilitation and augmentation. But on the other hand, there are also concerns about the possibility of the synthetic neurons growing uncontrollably and turning us all into brain-eating zombies. Okay, maybe that’s a bit of an exaggeration, but the point is that we must tread carefully in this field and thoroughly consider the potential risks and drawbacks of this technology.
Design of the FractalSpeak Neural Interface
In this section, we’re going to take a deep dive into the nitty-gritty of the FractalSpeak neural interface, specifically, the different designs that are currently being researched. First up, we have the “carpet neurode.” Imagine a shag carpet, but instead of fibers, it’s made up of a matrix of synthetic neurons. The key feature of this design is that the digitations, or projections, are of varying lengths, allowing for a more randomized and natural distribution in the brain tissue.
Next, we have the “bio array neurode.” This design is all about precision. It’s shaped like a sphere and each layer sends its projections to specific lengths, creating an electrical image of the tissue it’s in contact with. It’s like a 3D map of the brain, but instead of streets and buildings, it’s made up of neural connections.
Last but not least, we have the “tesseract neurode.” This design is inspired by the geometric shape of a tesseract. Each layer is growth-fixed and sends its projections to specific lengths within the tissue. The cells of different grids are interconnected with specific rules, forming a rich network of neural connections. It’s like building a neural city, with skyscrapers made of synthetic neurons.
The carpet neurode:
The “carpet neurode” design is a base model of an interwoven matrix that is composed of synthetic neuron-astrocyte hybrids. The synthetic neurons are located on the grids created by the electronic part of the carpet neurode, and the pattern of interdigitation is carefully designed to mimic the structure of the healthy brain tissue. The synthetic neurons are able to interdigitate into the healthy brain tissue, creating a seamless interface between the synthetic and biological layers. The synthetic neurons are able to gather neuronal information by sending out digitations of various lengths into the brain tissue, allowing for simultaneous data collection from different depths. This information is then stored in the synthetic layer and transferred to the artificial neural layer via the silicone interface. This allows for a more detailed and accurate representation of the brain tissue, increasing the resolution and amount of data that can be stored. One of the major advantages of this design is that it allows for a direct interaction between the synthetic and biological layers, allowing for a more efficient transfer of information between the two. Additionally, the interdigitations going deep inside the tissue also allows for the gathering of data from deeper regions of the brain, which would otherwise be difficult to access. However, there are also some challenges that need to be overcome with this design. One of the main challenges is the risk of dehiscence, where the carpet neurode may shift or move after surgery. To mitigate this risk, an inflatable catheter is placed to keep the carpet neurode in place. Additionally, fractal geometry is used in the design to ensure a more stable and secure placement of the neurode.
Fractal geometry:
Fractal geometry can be used in the context of the carpet neurode design to drive and guide the growth of the synthetic neuronal layer into damaged brain tissue. This is achieved by incorporating fractal patterns into the design of the electronic grid that forms the base of the neurode. The fractal patterns are used to create a stable and secure placement of the neurode, reducing the risk of dehiscence.
Fractal geometry is important because it allows for the creation of complex and highly organized structures that can mimic the natural architecture of the brain. This is crucial in ensuring that the synthetic neuronal layer can integrate seamlessly into the healthy brain tissue. By mimicking the natural architecture of the brain, fractal geometry can also help to preserve the “inner state” of the biological network and improve the representation of neural information.
Furthermore, the use of fractal geometry in the design of the carpet neurode can have implications for synthetic biology. By incorporating fractal patterns, we can create synthetic structures that are able to mimic the complex and highly organized architecture of living systems. This can be beneficial in a range of applications, including tissue engineering, drug delivery, and biosensors.
In the study “Creating cellular patterns using genetically engineered, gold- and cell-binding polypeptides” the authors used a fractal inspired approach for the design of the patterned surfaces, which the cells adhere to, in order to achieve a high precision, fidelity, and stability in the patterning of cells. This approach could be applied to create a fractal patterned electronic grid in the carpet neurode design, which would increase the stability of the neurode and improve the representation of neural information.
In the study “Programming self-organizing multicellular structures with synthetic cell-cell signaling” authors used a synthetically engineered signaling system that programs cell-cell contact-dependent pattern formation. This approach could be used to drive the growth of the synthetic neuronal layer in the carpet neurode design, and guide it to interdigitate with the healthy brain tissue.
In summary, fractal geometry can be used in the carpet neurode design to drive and guide the growth of the synthetic neuronal layer into damaged brain tissue, by mimicking the natural architecture of the brain and preserving the “inner state” of the biological network. This can improve the representation of neural information and has implications for synthetic biology.
Different Cell Designs by DALL-E
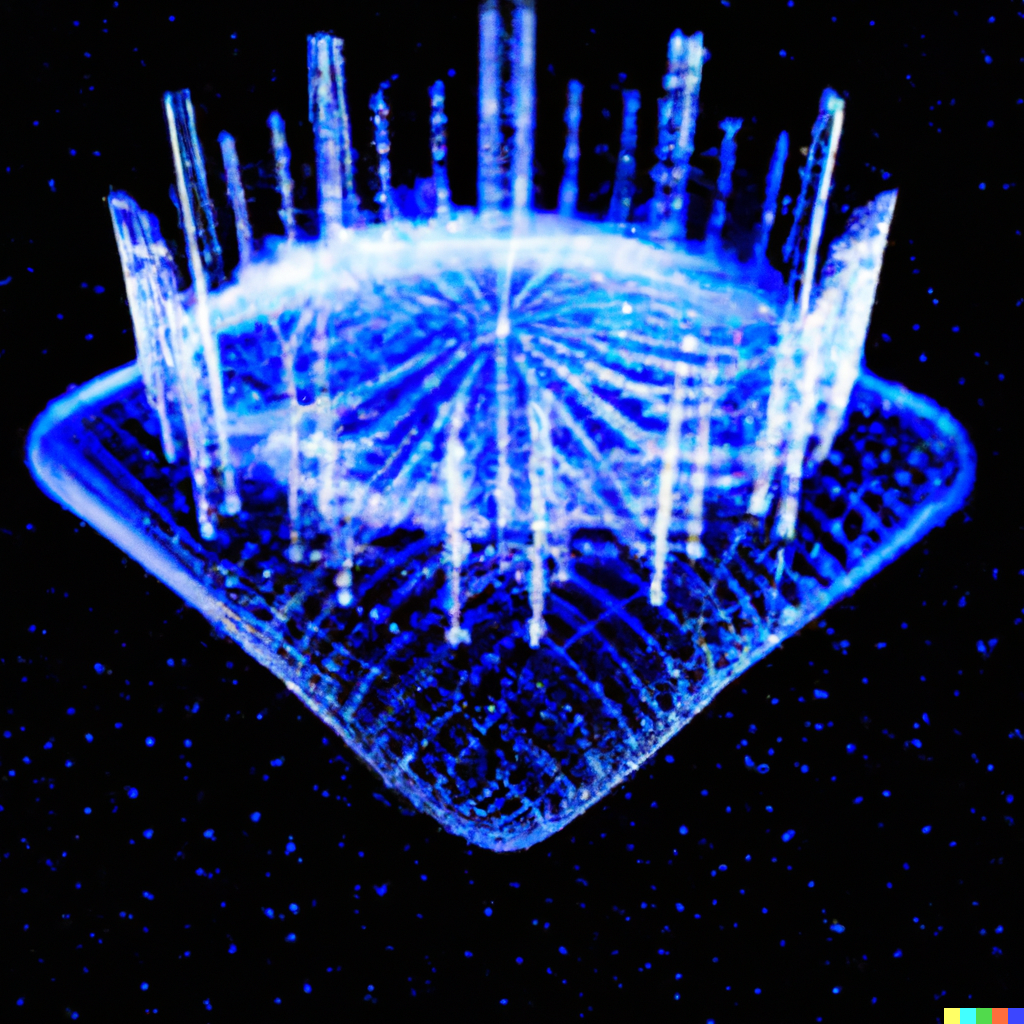
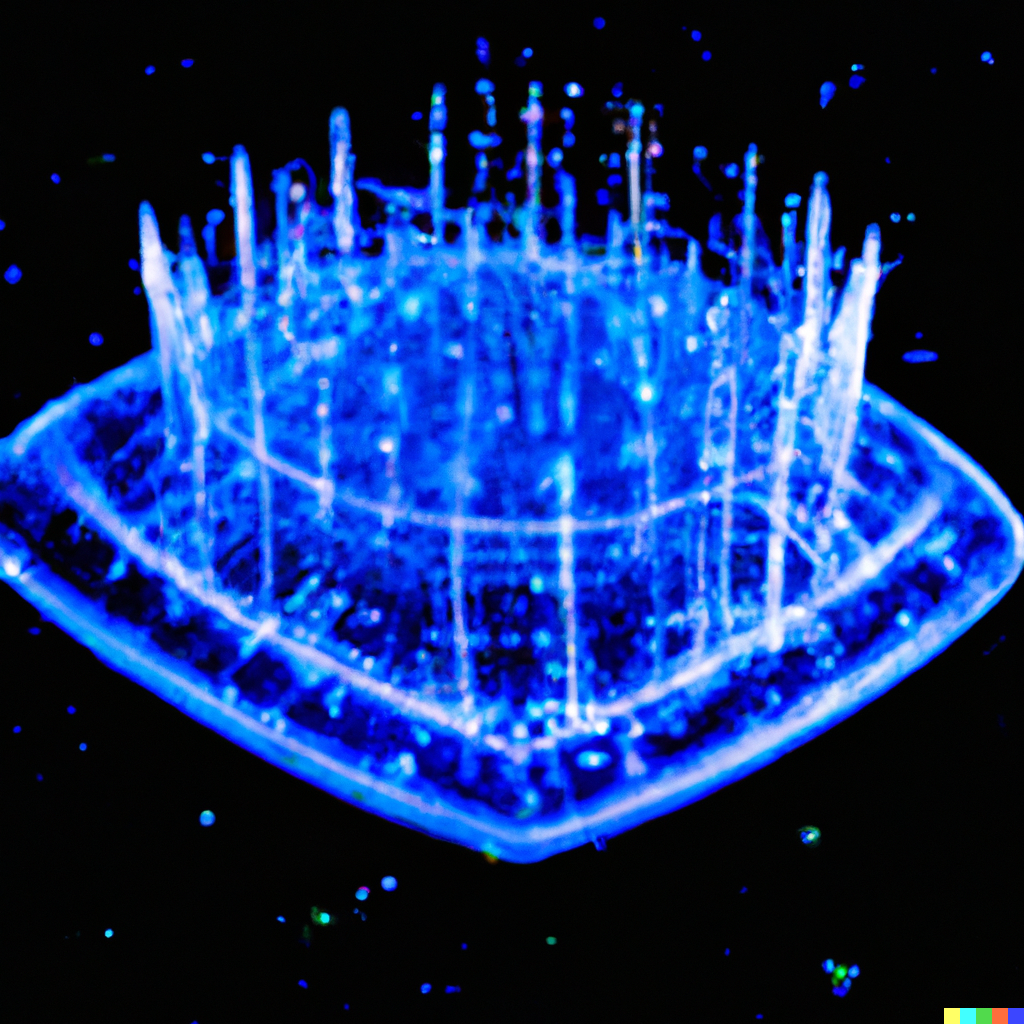
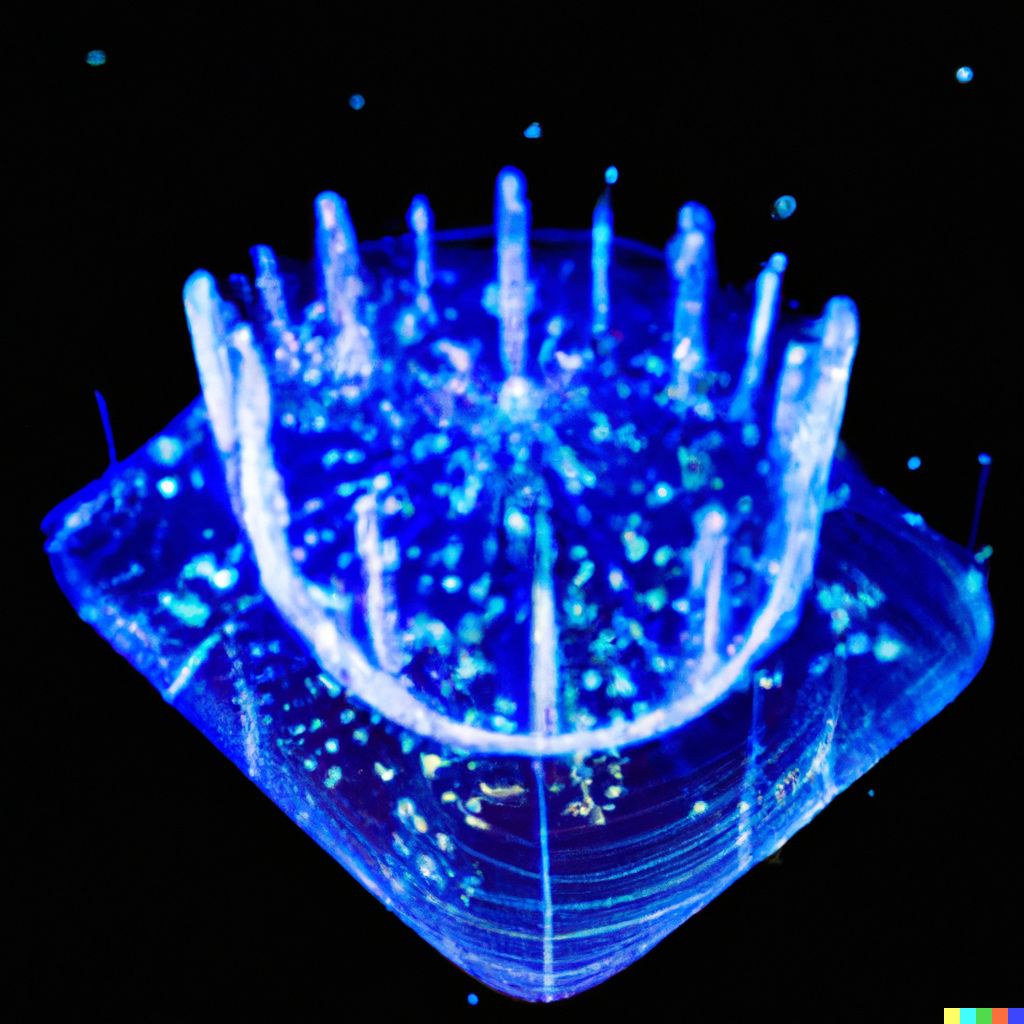
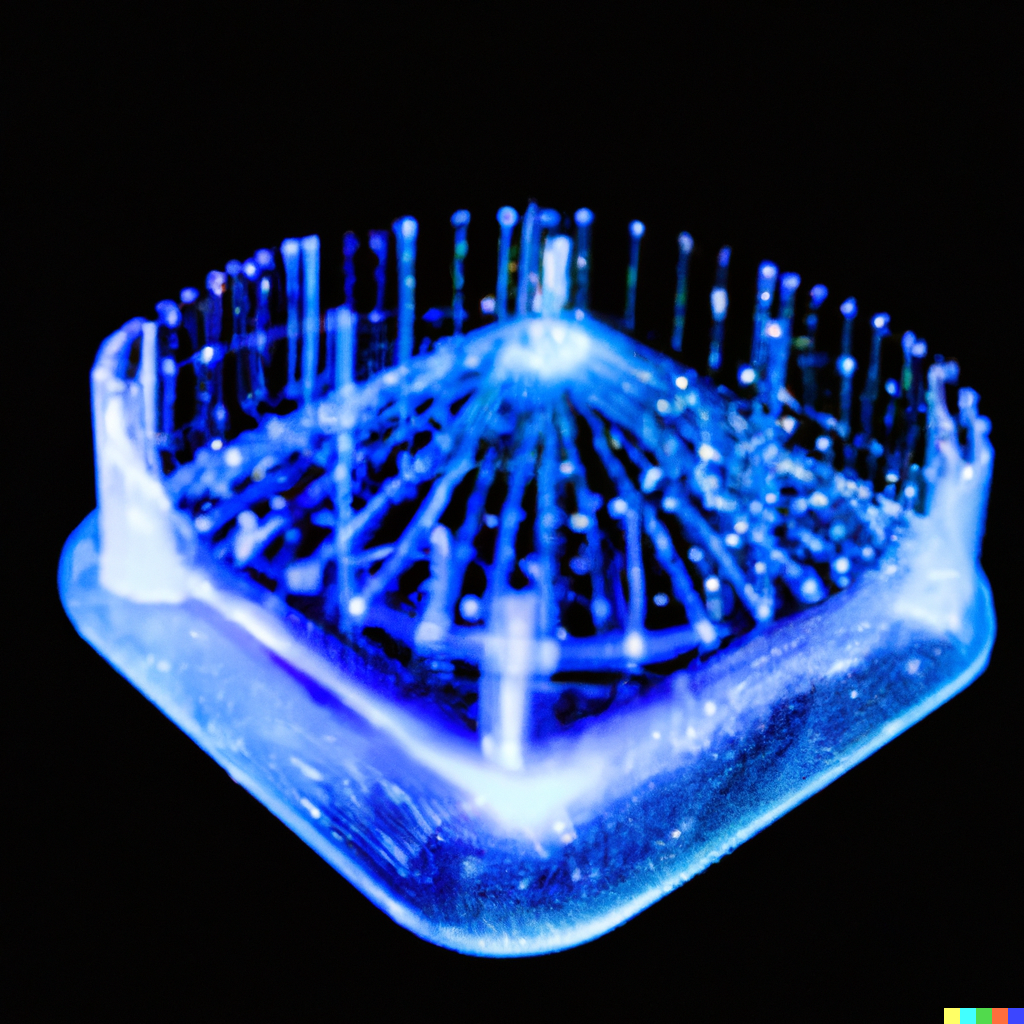
The bio array and tesseract neurodes:
As we’ve discussed, the “carpet neurode” design is a base model of an interwoven matrix that is composed of synthetic neuron-astrocyte hybrids. The synthetic neurons are located on the grids created by the electronic part of the carpet neurode, and the pattern of interdigitation is carefully designed to mimic the structure of the healthy brain tissue. However, there are other designs that have been proposed to improve upon this basic design.
One such design is the “bio-array neurode.” This design is similar to the carpet neurode, but with an additional layer. The bio-array neurode consists of multiple layers of synthetic neurons, each with different projections. These projections are able to interdigitate with the healthy brain tissue at different depths, allowing for a more detailed and accurate representation of the brain tissue. This increased resolution and amount of data that can be stored is an improvement over the carpet neurode design.
Another design is the “tesseract neurode.” The tesseract neurode is a more complex design that consists of multiple layers of synthetic neurons with different projections. The projections are able to interdigitate with the healthy brain tissue at different depths, similar to the bio-array neurode. The tesseract neurode also includes a fourth dimension, which allows for the gathering of data from even deeper regions of the brain. The tesseract neurode design allows for the most detailed and accurate representation of the brain tissue, but also the most complex and difficult to achieve.
Both the bio-array and tesseract neurode designs take advantage of fractal geometry to drive and guide the growth of the synthetic neuronal layer into damaged brain tissue. The fractal geometry allows for a more stable and secure placement of the neurode, which is particularly important for the deeper layers of the bio-array and tesseract neurode designs. Additionally, fractal geometry allows for a more detailed representation of the neural information, as it mimics the complex, self-similar structure of the biological network.
In conclusion, the “carpet neurode” design is a basic model of an interwoven matrix that is composed of synthetic neuron-astrocyte hybrids. However, there are other designs such as the “bio-array neurode” and “tesseract neurode” that have been proposed to improve upon this basic design. These designs take advantage of fractal geometry to drive and guide the growth of the synthetic neuronal layer into damaged brain tissue, allowing for a more detailed and accurate representation of the brain tissue. As the field of synthetic biology continues to advance, we can expect to see more complex and sophisticated designs that will ultimately lead to a better understanding and treatment of brain disorders.
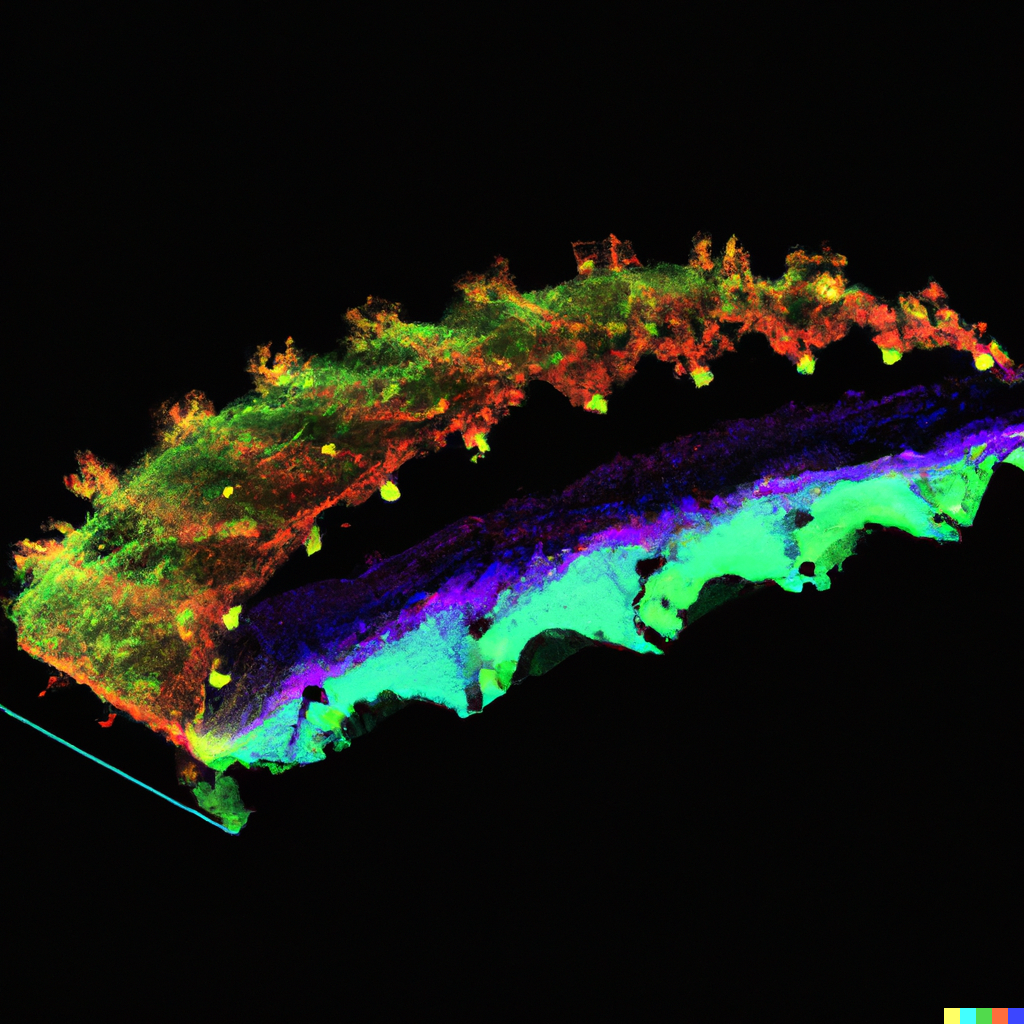
The docking process
Once the FractalSpeak neural interface has been successfully implanted in the damaged brain tissue, the next step is to establish a connection between the severed axons and the synthetic neurons in the interface. This process is known as “docking” and involves the use of specific neurochemicals to guide the severed axons towards the synthetic neurons.
The primary neurochemical involved in this process is called “netrin-1”. Netrin-1 is a guidance molecule that attracts axons towards it, and it is produced by the synthetic neurons in the FractalSpeak neural interface. The severed axons in the brain tissue are able to detect the presence of netrin-1 and are attracted towards it. This attraction is mediated by receptors on the axons called “deleted in colorectal cancer” (DCC) and “neogenin” which bind to netrin-1.
Once the severed axons are in close proximity to the synthetic neurons in the FractalSpeak interface, they make contact with the astrocytic projections that extend from the interface. These astrocytic projections are able to provide the severed axons with essential nutrients and anti-apoptotic molecules to ensure their survival. This is crucial for the long-term viability of the FractalSpeak interface.
In addition to netrin-1, other neurochemicals such as “neuregulin-1” and “slit-robo” have also been shown to play a role in the docking process. Neuregulin-1 is a protein that plays a critical role in the development and maintenance of synapses, while “slit-robo” is a family of proteins that are involved in axon guidance.
It is worth mentioning that the bio-array neurode and the tesseract neurode have different numbers of layers and projections, the bio-array neurode is designed with a single layer and a single projection, while the tesseract neurode is designed with multiple layers and projections. The number of layers and projections used in each design is dependent on the specific application and the degree of complexity required.
In summary, the docking of damaged axons to the FractalSpeak neural interface is a crucial step in the repair of damaged brain tissue. The use of specific neurochemicals such as netrin-1 and neuregulin-1, as well as astrocytic projections, help to guide the severed axons towards the synthetic neurons in the interface and ensure their survival. The number of layers and projections used in the design of the FractalSpeak neural interface is dependent on the specific application
The connections between synthetic layer and the silicone layer:
As we have discussed, the “carpet neurode” design is a base model of an interwoven matrix that is composed of synthetic neuron-astrocyte hybrids, designed to mimic the structure of healthy brain tissue. The synthetic neurons gather neuronal information by sending out digitations into the brain tissue, and this information is then stored in the synthetic layer and transferred to the artificial neural layer via the silicone interface. This allows for a more detailed and accurate representation of the brain tissue, increasing the resolution and amount of data that can be stored.
One of the key components of this design is the electro-chemical interactions between the synthetic layer and the electronic-silicone layer. The synthetic neurons are able to bind to the electronic-silicone layer through specific neurochemical interactions, allowing for communication between the two layers. This binding is facilitated by the use of specific messaging molecules, such as neurotransmitters and neuromodulators, which are able to bind to the electronic-silicone layer and transmit information between the synthetic and electronic layers.
The electronic-silicone layer is able to detect the specific neurotransmitters and neuromodulators released by the synthetic neurons, and is able to translate this information into an artificial image of the connections networks formed by the synthetic neuron-astrocyte hybrids. This image is then able to be interpreted by the electronic-silicone layer, allowing for a more accurate and detailed representation of the neural activity within the brain tissue.
This electro-chemical communication between the synthetic and electronic layers is crucial for the functioning of the carpet neurode, as it allows for the gathering of data from deeper regions of the brain and a more efficient transfer of information between the synthetic and biological layers. Additionally, this communication also allows for the monitoring of the “inner state” of the biological network, allowing for a more accurate understanding of the neural activity within the brain tissue.
In summary, the electro-chemical interactions between the synthetic layer and the electronic-silicone layer in the “carpet neurode” design are crucial for the gathering of data from deeper regions of the brain, and for the efficient transfer of information between the synthetic and biological layers. This communication allows for the monitoring of the “inner
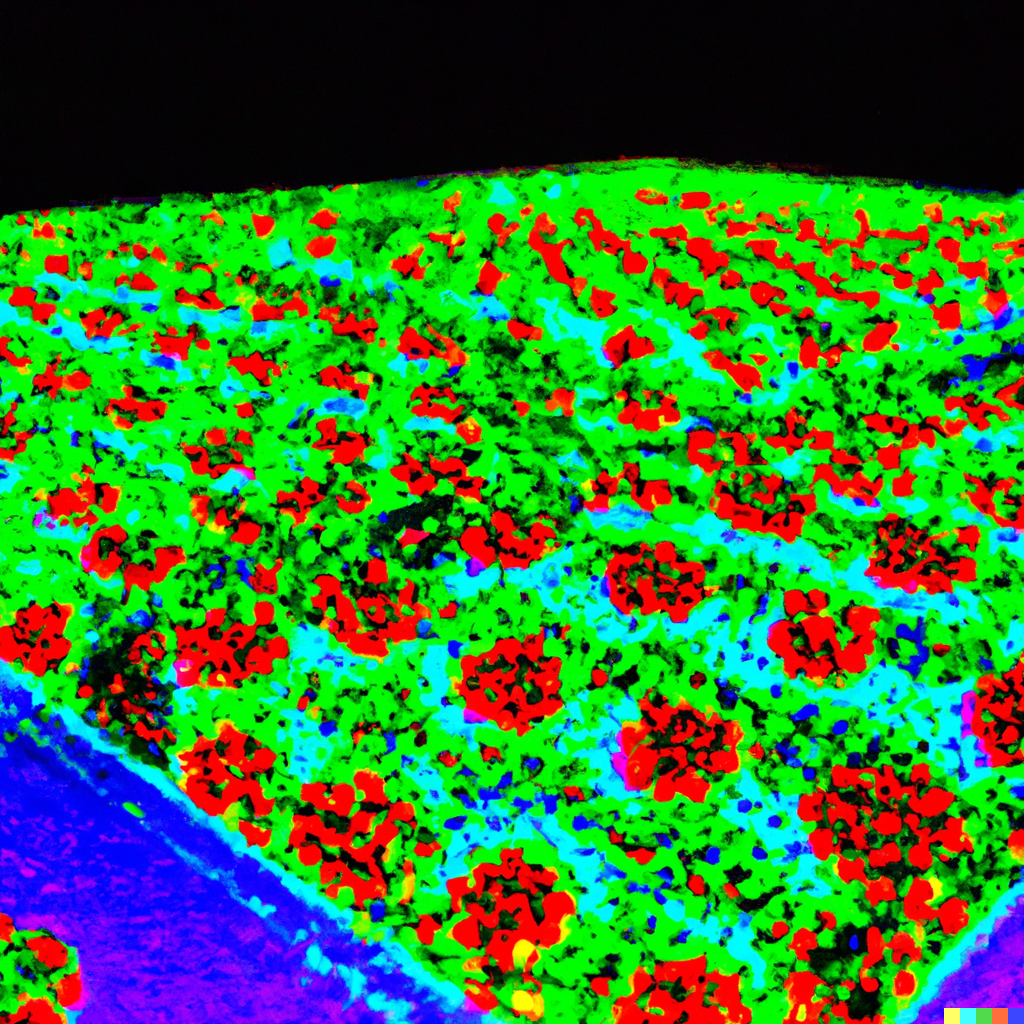
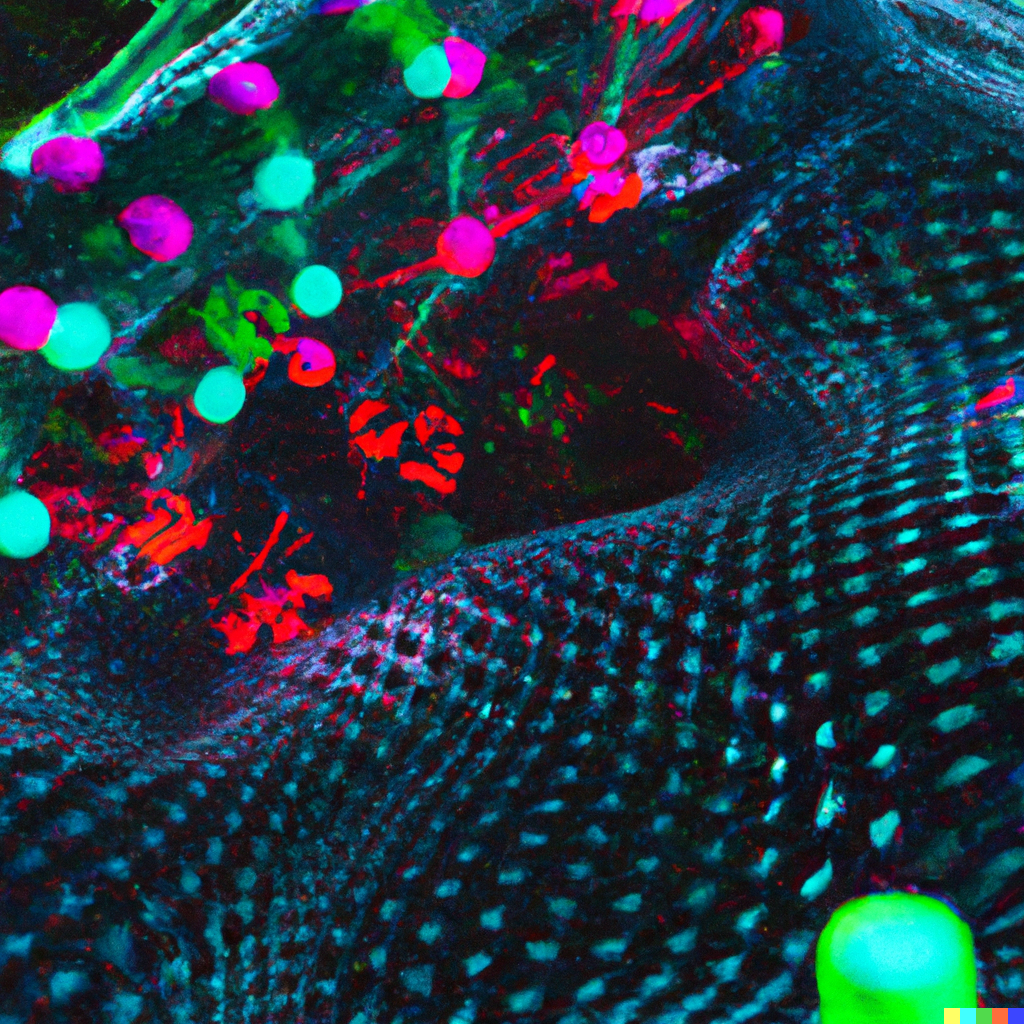
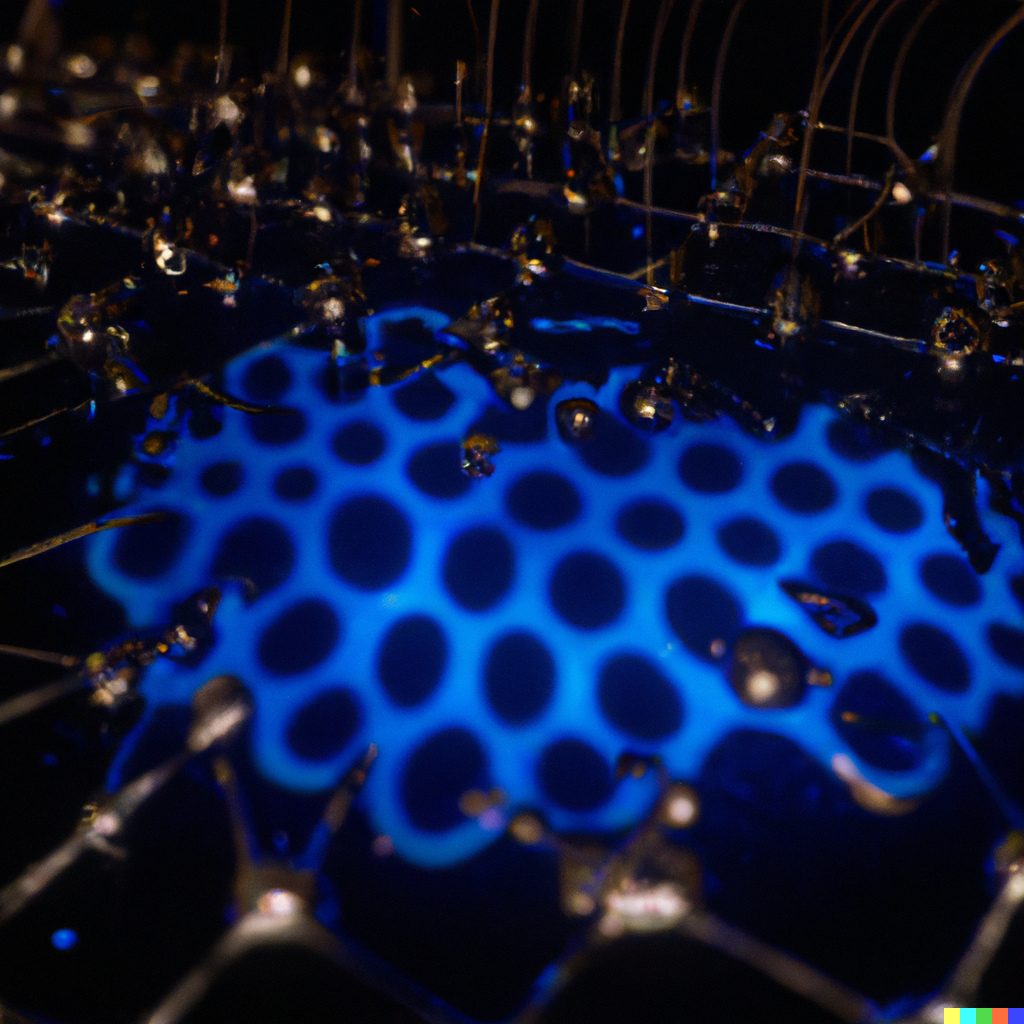
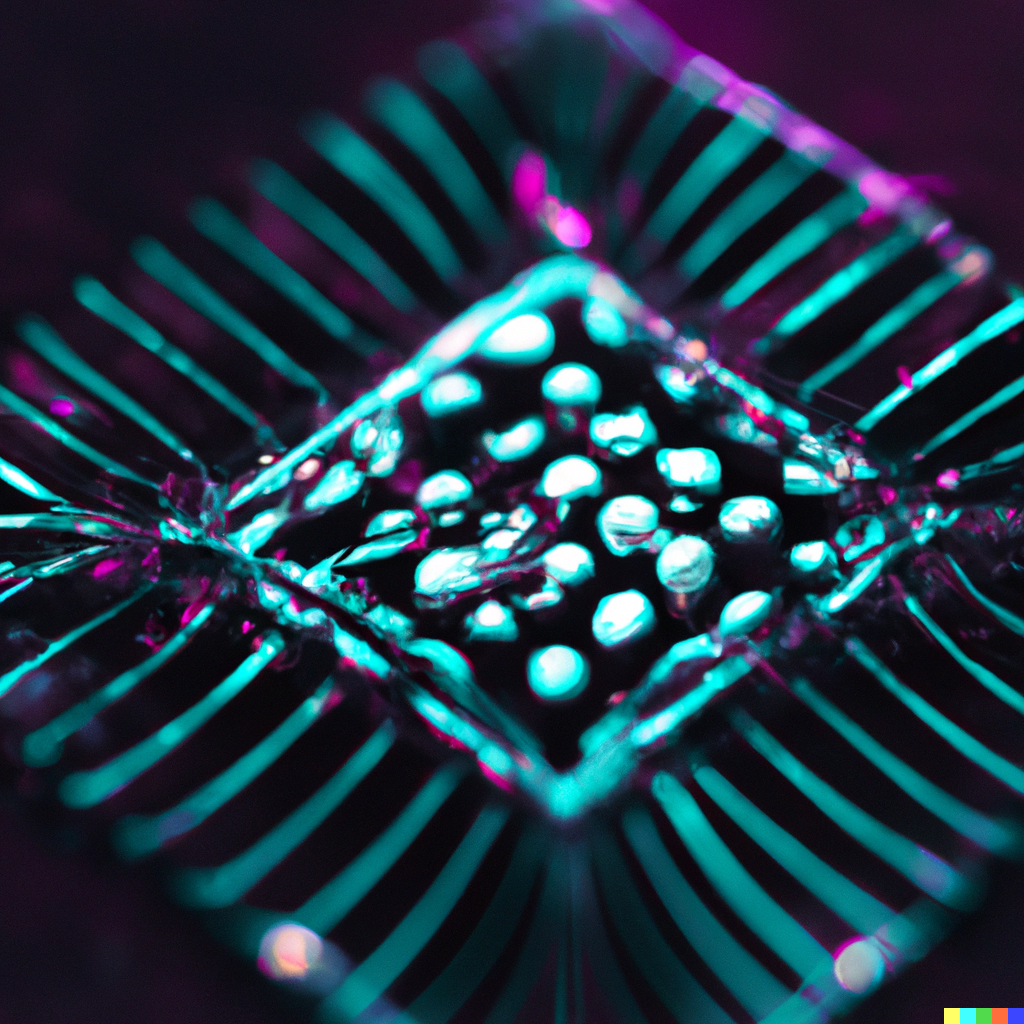
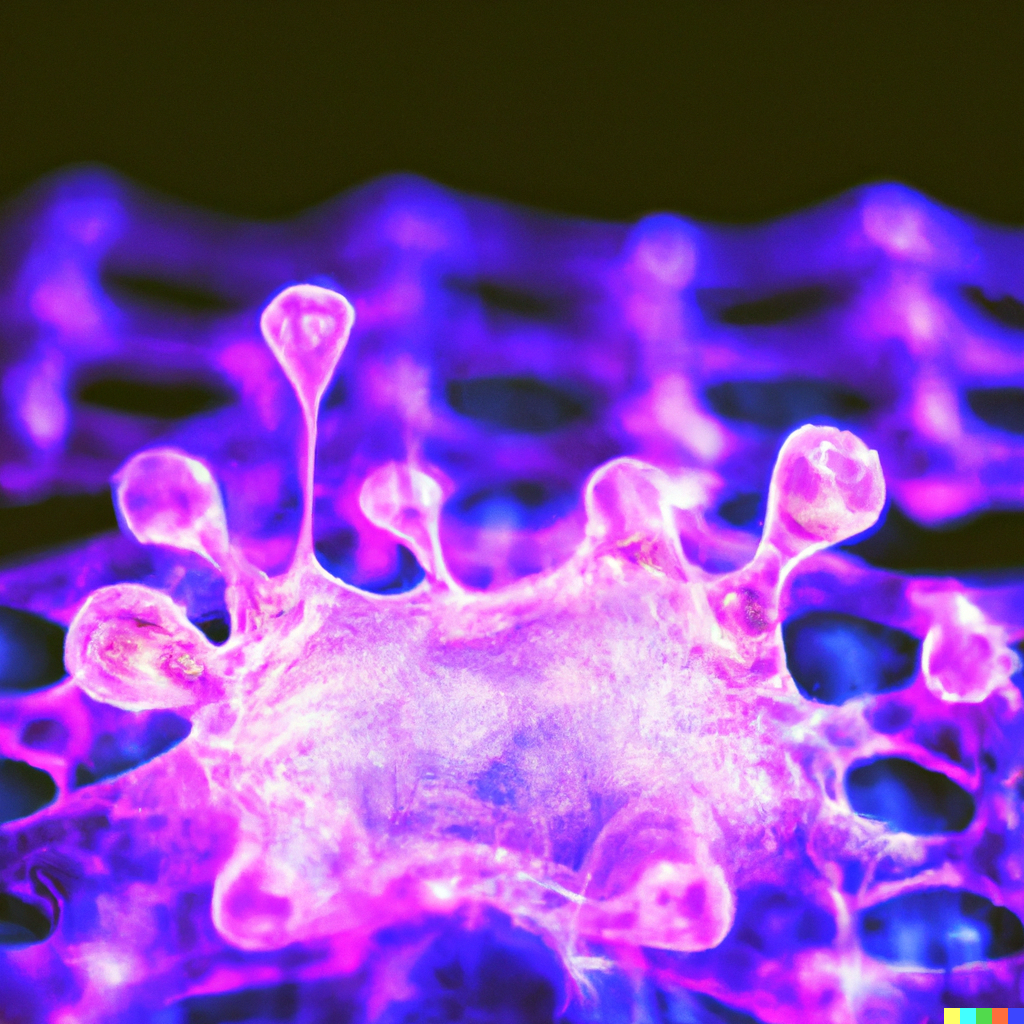
The use of astrocyte-neuron hybrid cells:
The creation of synthetic astrocyte-neuron hybrid cells is a crucial step in the development of the FractalSpeak neural interface. These cells are created using a combination of synthetic biology techniques, including genetic engineering and cell culture methods.
One of the key techniques used in the creation of these cells is the use of viral vectors to introduce specific genes into the cells. These genes encode for the proteins and other molecules necessary for the formation of the synthetic neuron-astrocyte hybrid cells. This method allows for precise control over the genetic makeup of the cells, ensuring that they possess the desired properties and functions.
Another technique used in the creation of these cells is the use of stem cells. These cells have the ability to differentiate into various types of cells, including neurons and astrocytes. By carefully controlling the conditions under which the stem cells are grown and differentiated, it is possible to create hybrid cells that possess the properties of both neurons and astrocytes.
We chose to create a neuron-astrocyte hybrid cell rather than utilizing two separate cells for a few reasons. Firstly, astrocytes are known to play a crucial role in the health and survival of neurons. By incorporating astrocytic properties into the synthetic neuron cells, we can ensure that they are better able to survive and function within the FractalSpeak neural interface.
Additionally, astrocytes also play a crucial role in the formation and maintenance of the extracellular matrix, which provides structural support for the neurons and facilitates communication between them. By incorporating astrocytic properties into the synthetic neurons, we can ensure that they are better able to form functional connections with the surrounding brain tissue.
In summary, the creation of synthetic astrocyte-neuron hybrid cells is a crucial step in the development of the FractalSpeak neural interface. This is achieved by the combination of synthetic biology techniques such as genetic engineering and stem cell culture methods. The use of a neuron-astrocyte hybrid cell ensures better survival and function of the synthetic cells within the interface, and better formation and maintenance of connections with the surrounding brain tissue.
The cells:
The synthetic astrocyte-neuron hybrid cells play a crucial role in the FractalSpeak neural interface. These cells are specifically designed to mimic the behavior and functions of both astrocytes and neurons in the brain. They are engineered to have the unique abilities of both cell types, such as the ability to provide electrical excitation and release anti-apoptotic chemicals, respectively.
One of the key advantages of using these hybrid cells is their ability to respond to certain chemicals released by damaged axons. These chemicals, called chemoattractants, act as a beacon to guide the synthetic cells to the site of injury. The synthetic cells are genetically engineered to have receptors that specifically bind to these chemoattractants, allowing them to locate and dock onto the damaged axons.
Once docked, the synthetic astrocyte-neuron hybrid cells provide important support to the damaged axons. The astrocytic projections of the synthetic cells release various neuroprotective molecules, such as growth factors and anti-apoptotic molecules, to promote the survival of the damaged axons. Additionally, the synthetic neurons release electrical impulses, which help to stimulate the damaged axons and facilitate their repair and regeneration.
In summary, the synthetic astrocyte-neuron hybrid cells play an important role in the FractalSpeak neural interface by providing crucial support to damaged axons. Their ability to respond to chemoattractants allows them to locate and dock onto the site of injury, where they can release essential neuroprotective molecules and electrical impulses to aid in the repair and regeneration of the damaged axons.
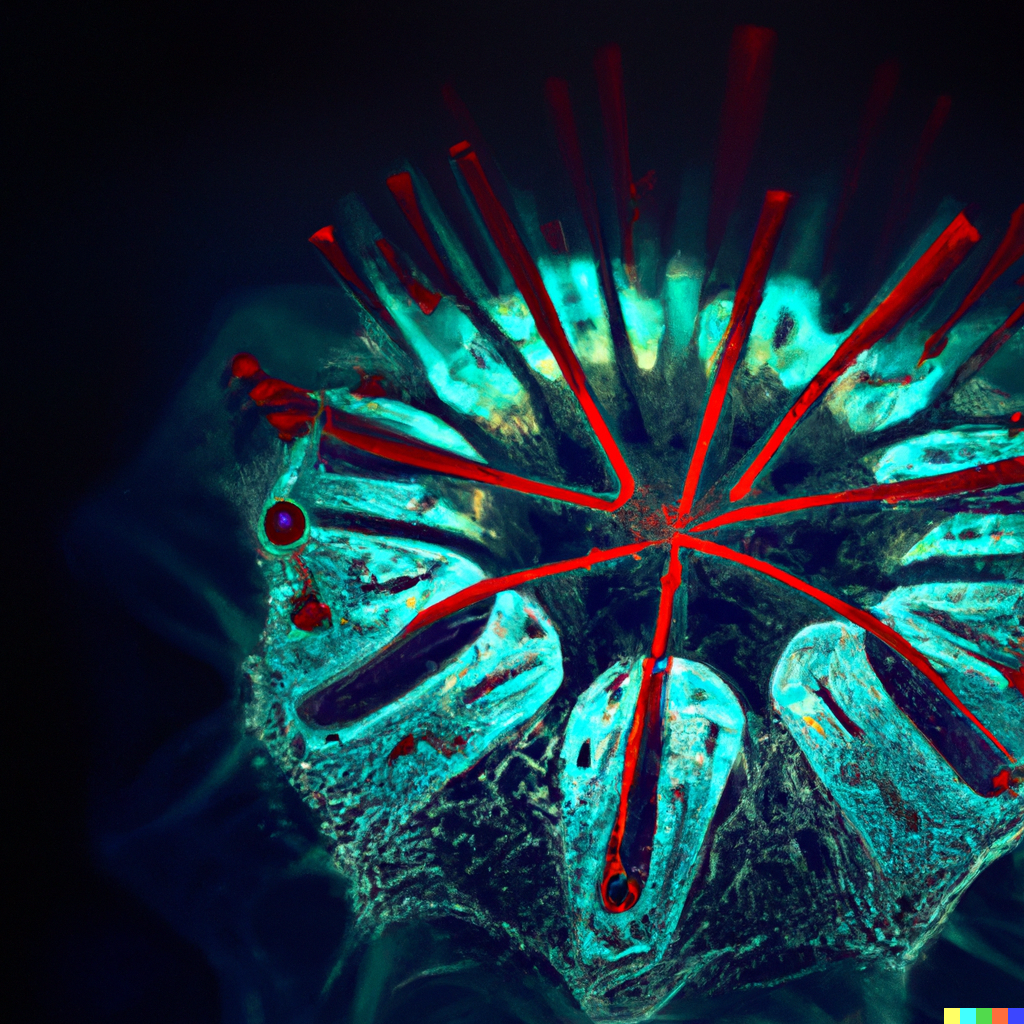
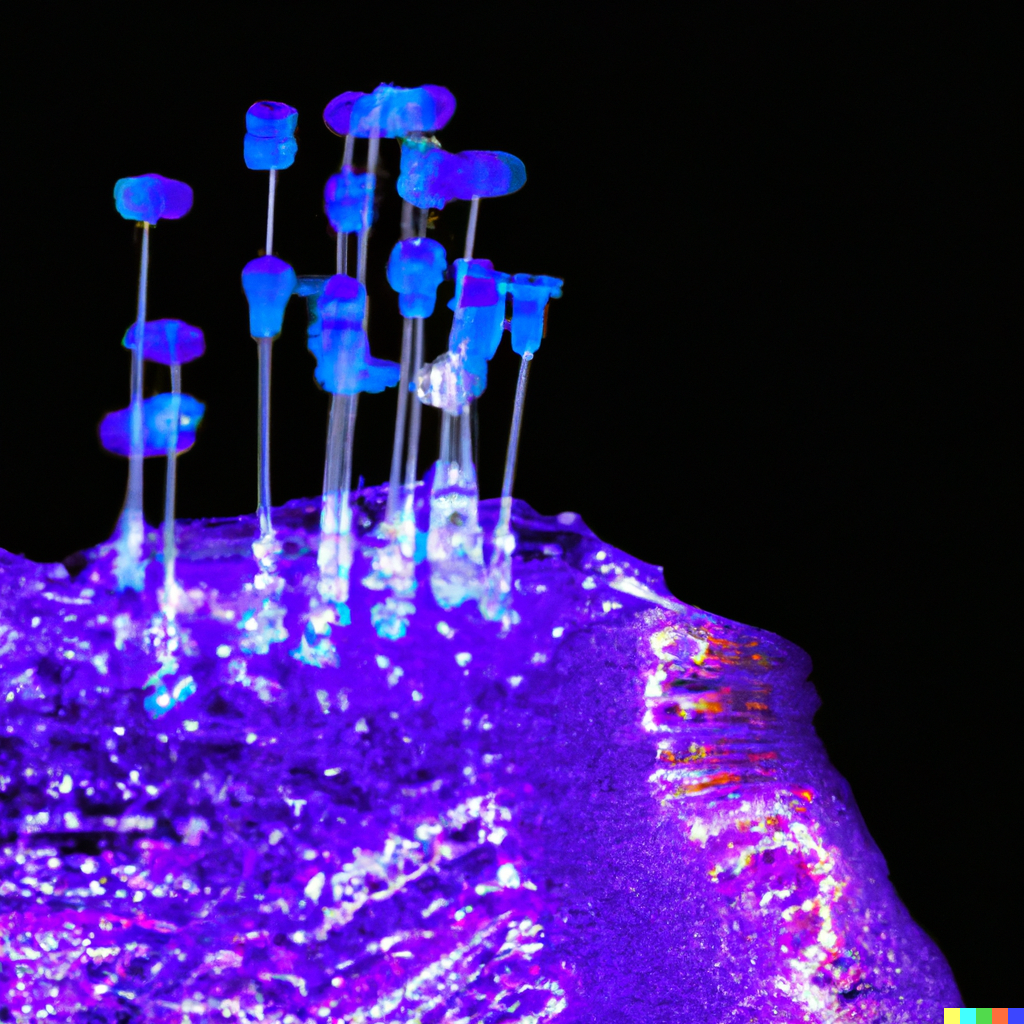
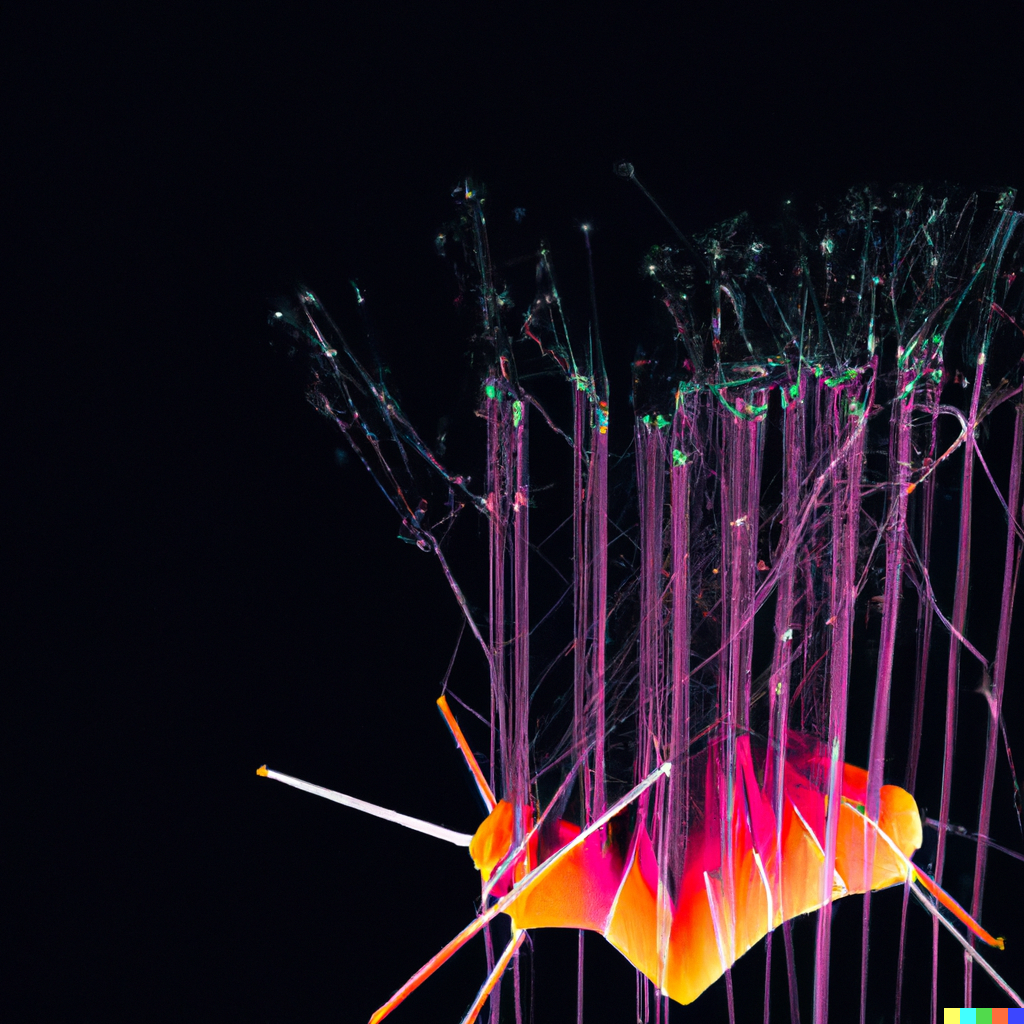
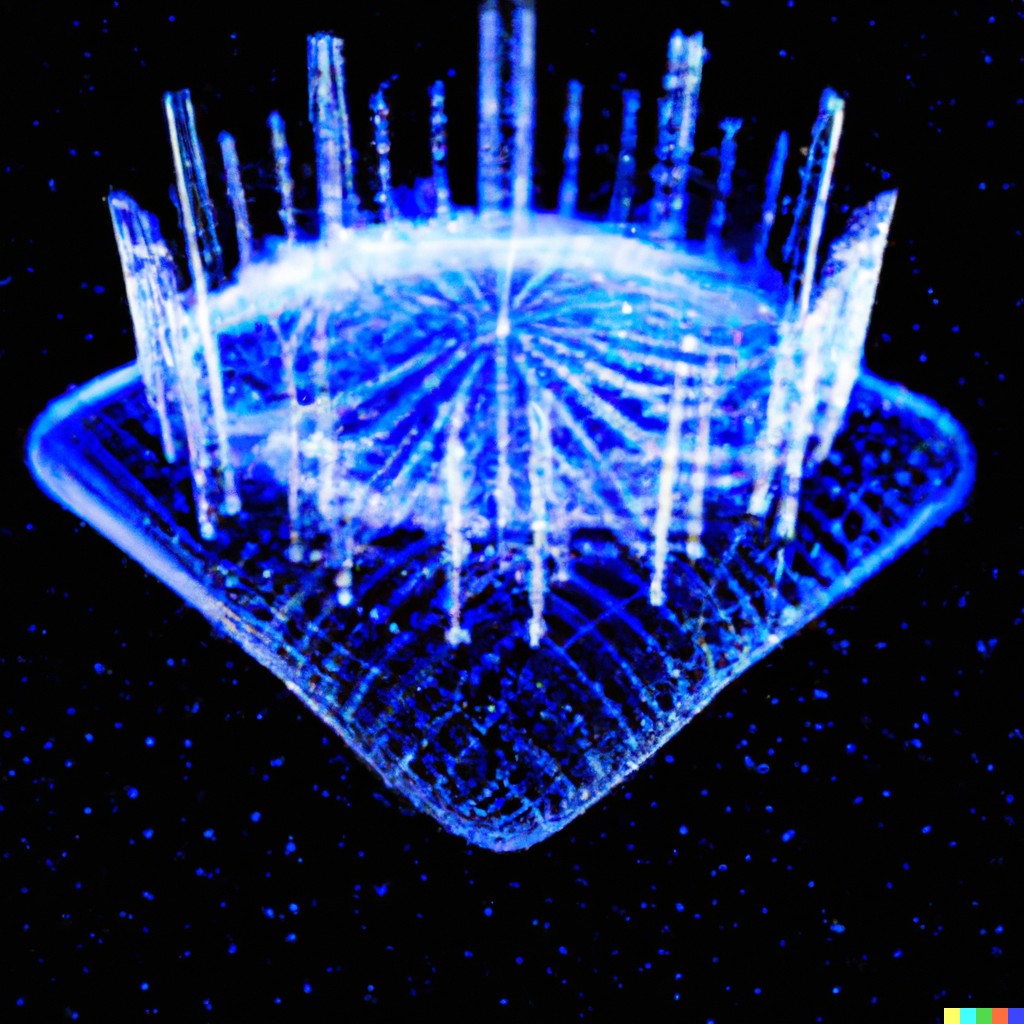
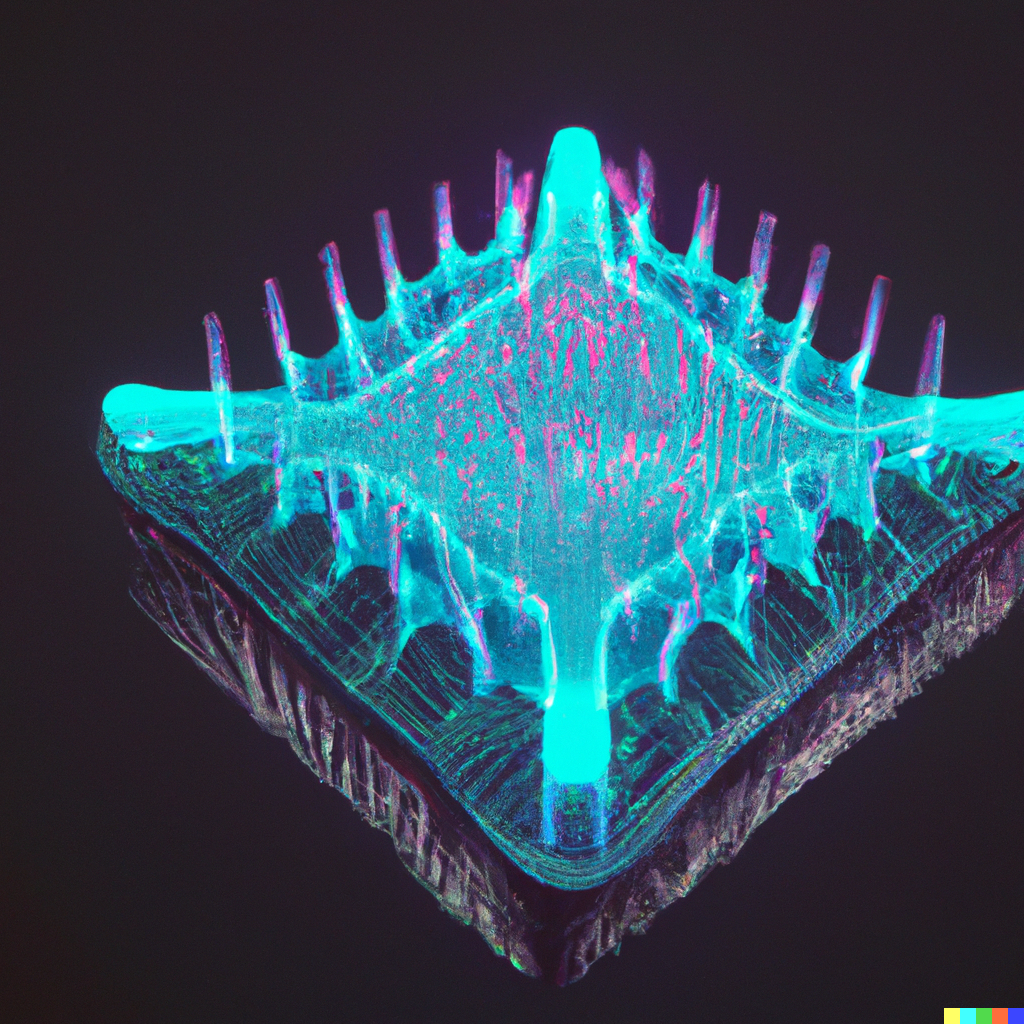
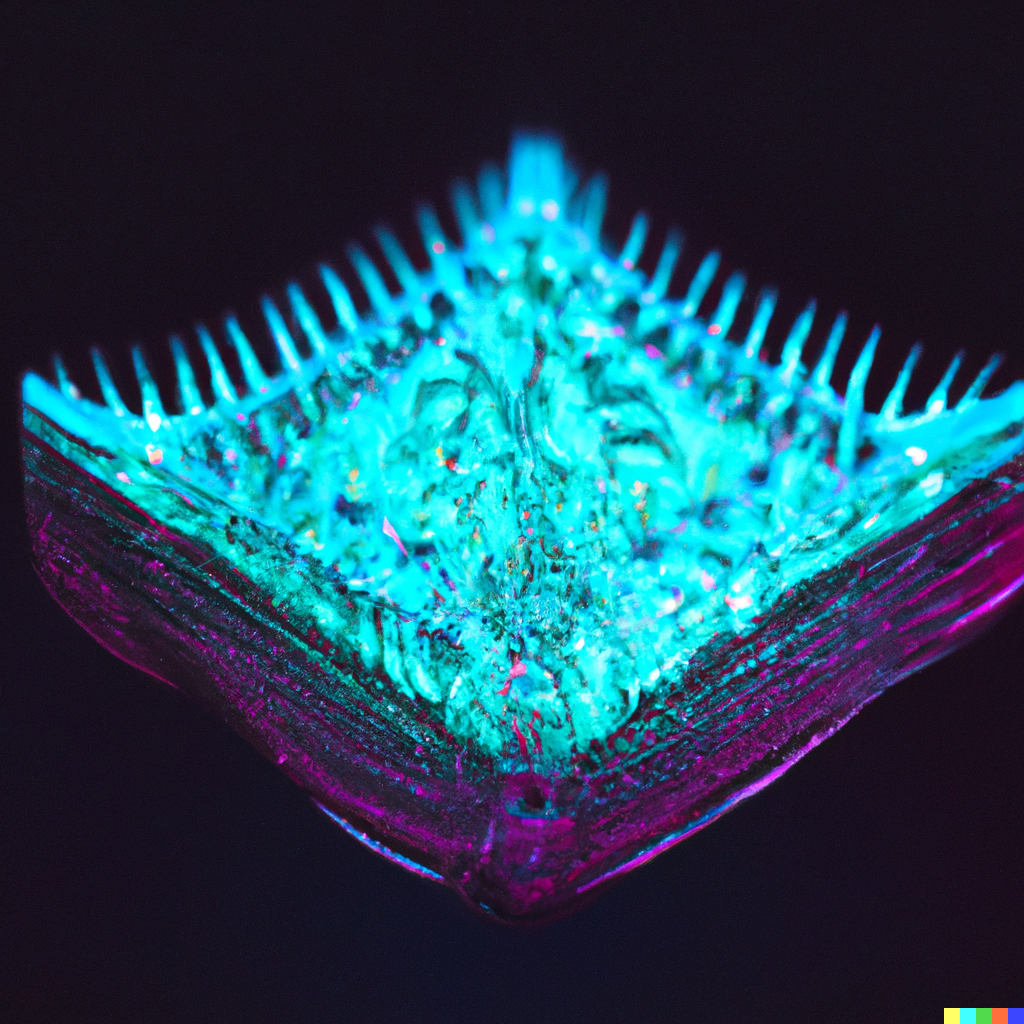
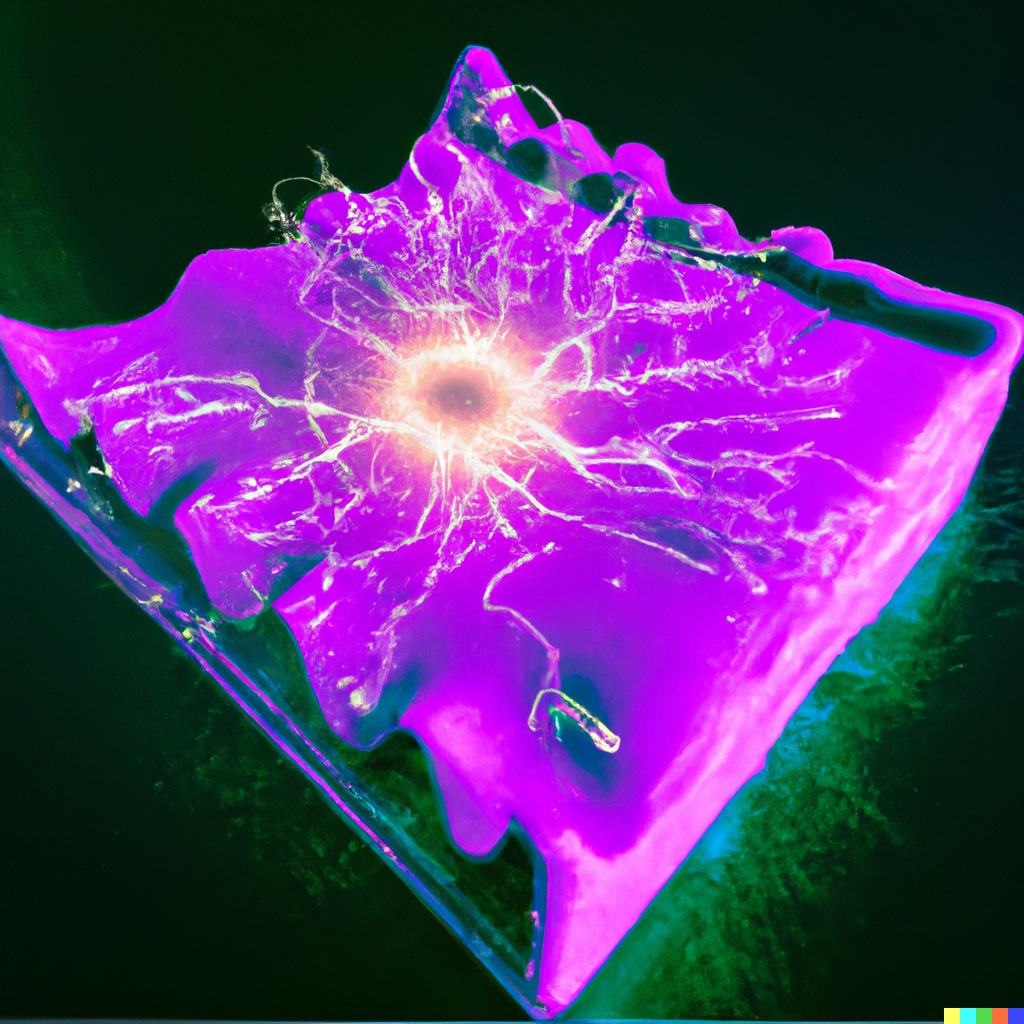
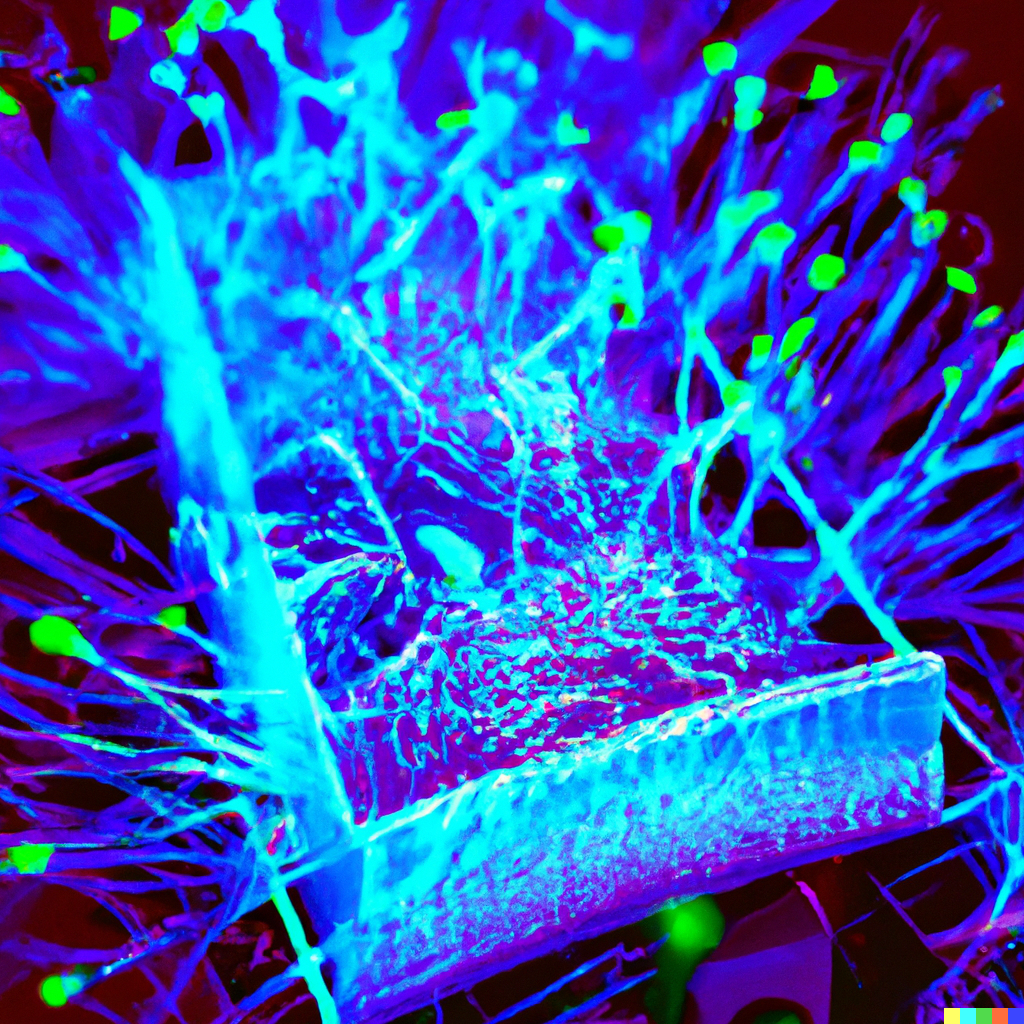
Different Dall-E conceptualizations for dendritic growth and digitation
Interconnectedness and Network-to-Network Communication
Introduction:
The concept of interconnectedness is crucial in the design and function of the FractalSpeak neural interface. This chapter will delve into the importance of interconnectedness in the FractalSpeak neural interface, as well as explore different ways to drive and measure interconnectedness in the synthetic layer and in the tissue. Additionally, we will investigate the potential for network-to-network communication between the FractalSpeak neural interface and the brain.
I. Importance of Interconnectedness:
Interconnectedness refers to the connections and interactions between different elements of a system. In the case of the FractalSpeak neural interface, interconnectedness refers to the connections between the synthetic layer and the tissue, as well as the communication between the synthetic neurons and astrocytes.
Interconnectedness is crucial for the FractalSpeak neural interface because it enables seamless communication and data transfer between the synthetic and biological layers. By creating a network of connections between the synthetic layer and the tissue, the FractalSpeak neural interface is able to gather a more detailed and accurate representation of the brain tissue, increasing the resolution and amount of data that can be stored.
Additionally, interconnectedness is crucial for the proper functioning of the FractalSpeak neural interface. The synthetic neurons and astrocytes need to be able to communicate and interact with each other, as well as with the tissue, in order for the FractalSpeak neural interface to properly function. Without interconnectedness, the FractalSpeak neural interface would not be able to effectively gather and transfer information, and would ultimately not be able to achieve its goal of facilitating communication between the brain and generative AI.
IV. Interconnectedness and Network-to-Network Communication
Introduction:
The FractalSpeak neural interface is designed to seamlessly integrate with the human brain, and one of the key factors in achieving this is through interconnectedness. In this section, we will explore the different ways in which interconnectedness is achieved and maintained in the FractalSpeak neural interface. We will also investigate the potential for network-to-network communication between the FractalSpeak neural interface and the brain.
Driving and Measuring Interconnectedness in the Synthetic Layer and Tissue:
One of the ways in which interconnectedness is achieved in the FractalSpeak neural interface is through the use of interdigitations created by the synthetic layer. These interdigitations are designed to mimic the structure of healthy brain tissue and provide a more detailed and accurate representation of the brain tissue. They act as a bridge between the synthetic and biological layers, allowing for a more efficient transfer of information between the two.
The interdigitations created by the synthetic layer also increase the bandwidth and resolution of the FractalSpeak neural interface. This is achieved by allowing the synthetic neurons to gather neuronal information by sending out digitations of various lengths into the brain tissue, thereby allowing for simultaneous data collection from different depths. This increases the amount of data that can be stored and improves the resolution of the
FractalSpeak neural interface.
Fractal geometry, network science, and manifolds can play a significant role in representing the interconnectedness between cells in the synthetic layer of the FractalSpeak neural interface. By using fractal geometry, the synthetic layer can be designed to mimic the intricate branching patterns found in the neural networks of the brain. This allows for the creation of a large number of interconnections between the synthetic neurons and the surrounding brain tissue, increasing the bandwidth and resolution of information exchange.
In addition to fractal geometry, network science can also be used to model the connectivity between the synthetic neurons and the biological tissue. By analyzing the topological properties of the synthetic neural network, researchers can gain a deeper understanding of how the synthetic neurons interact with the surrounding tissue. This can help identify areas where the synthetic neurons need to be more densely packed or where the interconnections need to be strengthened in order to improve the overall performance of the FractalSpeak neural interface.
Manifolds can also be used to represent the interconnectedness between the cells in the synthetic layer. Manifolds are a mathematical concept that can be used to describe the shape of a complex object. By representing the synthetic neural network as a manifold, researchers can gain a better understanding of how the network is structured and how it interacts with the surrounding tissue. This can help identify areas where the synthetic neurons need to be more densely packed or where the interconnections need to be strengthened in order to improve the overall performance of the FractalSpeak neural interface.
Overall, the use of fractal geometry, network science, and manifolds can help researchers better understand the interconnectedness between the cells in the synthetic layer, and how it can be used to improve the overall performance of the FractalSpeak neural interface. This can be particularly useful for representing the neuronal structure and information exchange in the FractalSpeak neural interface, as it allows for a more detailed and accurate representation of the neural networks in the brain.
GENERATIVE MODELS
In the realm of Artificial Intelligence, generative models have emerged as a powerful tool for various applications such as speech synthesis, image and text generation, and natural language processing. These models are trained on large datasets to learn the underlying patterns and generate new, previously unseen samples. In the context of FractalSpeak neural interfaces, generative AI models hold great potential for the rehabilitation of aphasic patients, who have lost the ability to speak as a result of brain injury or disease.
Aphasia is a communication disorder that affects an individual’s ability to produce and understand language. This condition can be caused by a stroke, traumatic brain injury, or degenerative diseases such as Alzheimer’s. Aphasia can have a devastating impact on an individual’s quality of life, affecting their ability to interact with others, work, and live independently.
Traditionally, speech therapy has been the primary treatment for aphasia. However, with the advancement of technology, researchers are now exploring the use of generative AI models as an alternative treatment. These models have the ability to analyze speech patterns and generate new speech, which could potentially be used to help aphasic patients regain their ability to communicate.
In this chapter, we will explore the application of generative AI models in the context of FractalSpeak neural interfaces for the rehabilitation of aphasic patients. We will discuss the current state of the art in generative AI models, their potential challenges and limitations, and the opportunities for future research and development in this area.
In recent years, generative AI models have made significant advancements in the field of artificial intelligence. One of the most notable areas of progress has been in the realm of natural language processing, specifically in the generation of human-like speech. The development of models such as GPT-3 and BERT have shown the ability to produce highly coherent and fluent speech, even in the absence of explicit training data. These models are based on a deep learning architecture known as a transformer, which is capable of handling large amounts of data and learning complex relationships between input and output.
While the current state of the art in generative AI models has shown great promise, there are still many challenges that need to be addressed before these models can be used in real-world applications. One of the main challenges is the need for a large amount of high-quality training data, which can be difficult and expensive to obtain. Additionally, these models are computationally intensive and require powerful hardware to run effectively. Furthermore, the interpretability of these models remains a challenge, making it difficult to understand how a model is making its predictions. Despite these challenges, researchers continue to make progress in the development of generative AI models, and their potential applications in speech generation from aphasic patients is a promising area of research.
Generative ai and coupling with neural tissue:
In generative AI models, the architecture of the artificial neural network plays a crucial role in determining the output. One of the most popular architectures used in generative models is the Generative Adversarial Network (GAN). GANs consist of two neural networks, the generator and the discriminator. The generator network creates new data samples, while the discriminator network tries to distinguish between the generated samples and the real data. Through this adversarial process, the generator network learns to create realistic samples that are indistinguishable from real data.
Another popular architecture used in generative models is the Variational Autoencoder (VAE). VAEs consist of an encoder and a decoder network. The encoder network takes in the input data and maps it to a lower-dimensional representation, called the latent space. The decoder network then takes this latent representation and maps it back to the original data space. By training the VAE, the encoder network learns to find a useful representation of the input data in the latent space, allowing for the generation of new data samples by sampling from this space.
In the context of speech generation from aphasic patients, the input to these generative models would be the neuronal activity patterns recorded from the biological neural network. The goal would be to train the generative model to learn the relationship between these neuronal patterns and speech, allowing it to generate speech output based on the recorded neuronal activity. This would require the use of large datasets of neuronal activity patterns recorded from individuals with aphasia, as well as the development of specialized algorithms to process and analyze these complex data sets.
Manifolds and fractals in this context:
Fractal geometry and manifolds can be utilized in the coupling of the outputs of the neuronal tissue to the inputs of the generative neural network by providing a way to represent the complex, non-linear structure of the neuronal tissue in a computationally tractable manner. One way to do this is by using fractal geometry to model the self-similarity and hierarchical structure of the neuronal tissue, which can be used as a basis for creating a manifold representation of the neural data. This manifold representation can then be used as the input for the generative neural network, allowing it to learn patterns and features in the neural data that correspond to speech patterns.
Additionally, the use of fractal geometry and manifolds can also be used to create a more robust and accurate representation of the neural data, which can improve the performance of the generative neural network. This can be achieved by using techniques such as manifold alignment, which can align the neural data with the generative model, allowing for better feature extraction and representation.
Manifold aligning is a technique that is used to align the topology of the artificial neural network with that of the biological neural network. This is achieved by using techniques from differential geometry, such as principal component analysis, to map the high-dimensional inputs from the biological neural network onto a lower-dimensional manifold. This manifold is then used as the input space for the artificial neural network, which can then generate speech based on this input. The use of manifold aligning allows for the neural network to better understand the structure of the biological neural network and thus generate more accurate speech output. Additionally, by using fractal geometry, the neural network can also take into account the self-similarity and hierarchical structure of the biological neural network, which can further improve the accuracy of the speech output.
Ultimately, the use of fractal geometry and manifolds in the coupling of the outputs of the neuronal tissue to the inputs of the generative neural network can enable a more accurate and robust representation of the neural data, which can lead to improved performance and functionality in the generative model.
Describe the surgical procedures for implanting the FractalSpeak neural interface in the brain
The surgical procedure for implanting the FractalSpeak neural interface in the brain involves several steps. First, the patient is placed under general anesthesia and a small incision is made in the skull to access the brain. Next, using advanced imaging techniques such as MRI or CT, the surgeon identifies the specific area of the brain that needs to be targeted. Once the target area is identified, the surgeon carefully implants the FractalSpeak neural interface, being sure to avoid damaging any surrounding neural tissue.
The FractalSpeak neural interface is designed to be as minimally invasive as possible, so the size of the incision can be kept small. However, due to the delicate nature of the brain and the need for precision in implanting the device, the procedure can take several hours to complete. After the implant is in place, the surgeon will close the incision and the patient will be closely monitored for any complications during the recovery period.
It’s important to note that the surgical procedures are not yet in use, but are based on the current state of the art in the field.
It’s also important to note that the surgical procedure is not without risks. The brain is a delicate organ and even small mistakes during the implantation process can result in serious complications. In addition, as with any surgical procedure, there is a risk of infection, bleeding, and other complications during the recovery period.
Ethical Considerations
One of the main ethical considerations surrounding the development and use of the FractalSpeak neural interface is the question of autonomy and privacy. While the technology has the potential to greatly improve the lives of those suffering from neurological disorders, it also raises concerns about the control and access to personal information. For example, the ability to directly interface with a person’s brain raises the question of who has access to that information and how it may be used. Additionally, the possibility of misuse or abuse of the technology, such as using it for mind control or surveillance, must also be considered.
Another important ethical consideration is the potential for unintended consequences. While the FractalSpeak neural interface has the potential to improve the lives of those suffering from neurological disorders, it also raises questions about the long-term effects of the technology on the brain and its ability to integrate with the person’s overall sense of self. Additionally, the development and use of the technology must take into account the potential for unintended consequences for society as a whole, such as the potential for exacerbating existing inequalities.
As with any new technology, the development and use of the FractalSpeak neural interface must be guided by a careful consideration of the potential ethical implications. It is important to continue to engage in open and transparent dialogue about these issues as the technology develops and evolves in order to ensure that its benefits are realized while minimizing any negative impacts.
Some other cool images not included in the text, generated by Dall-E
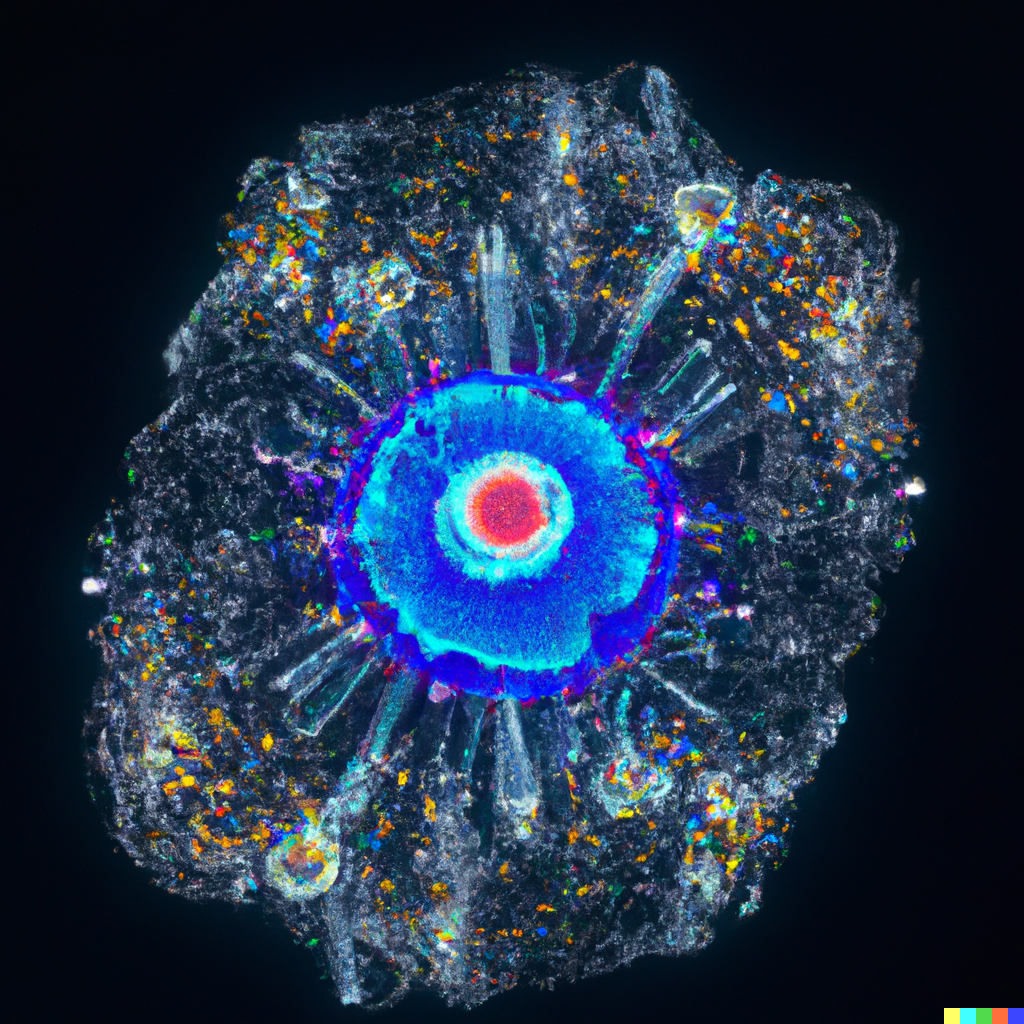
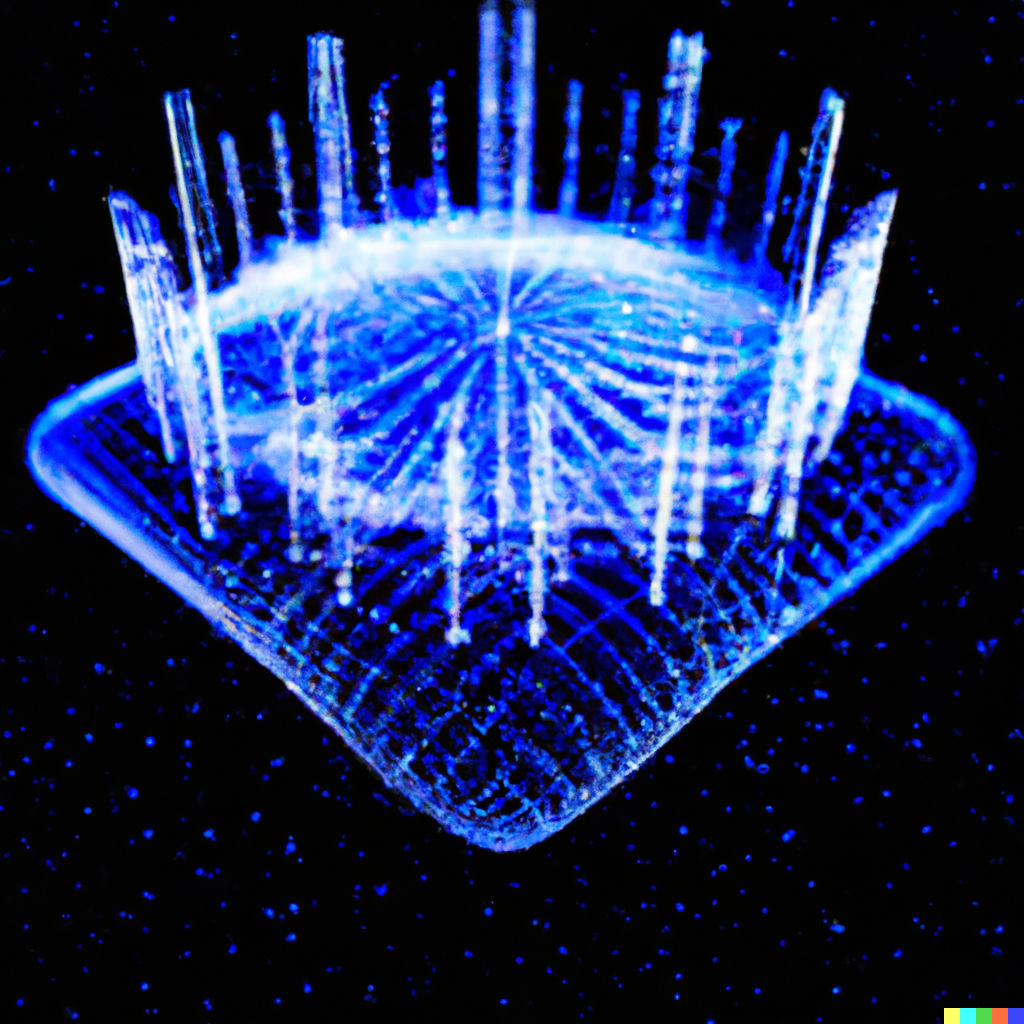
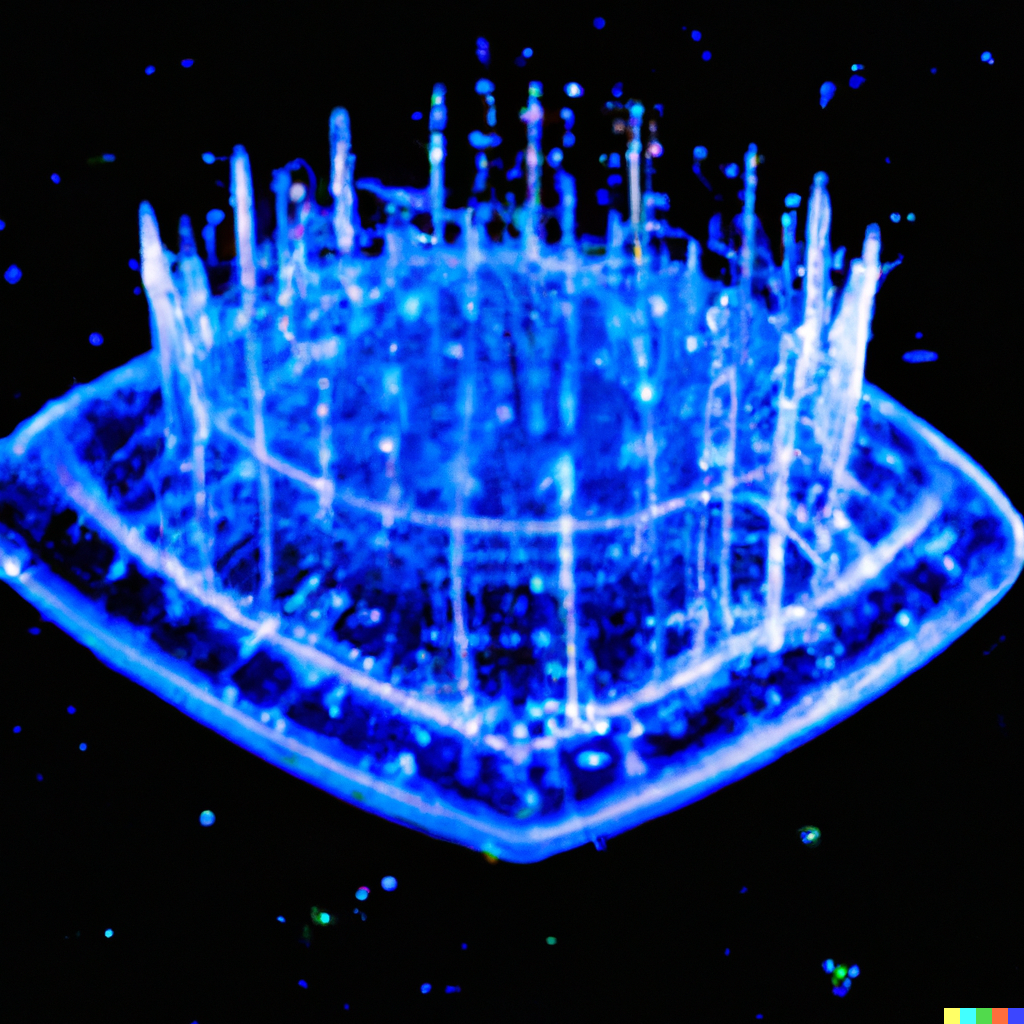
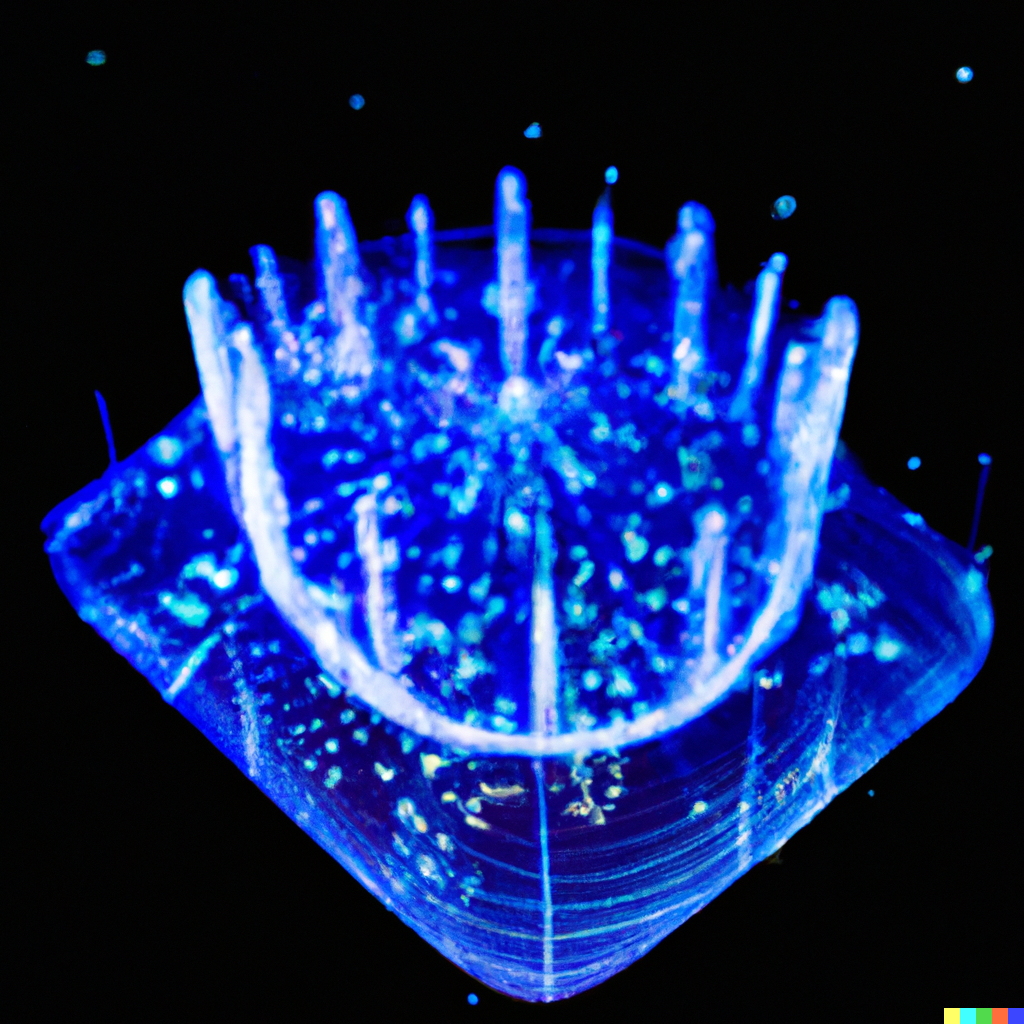
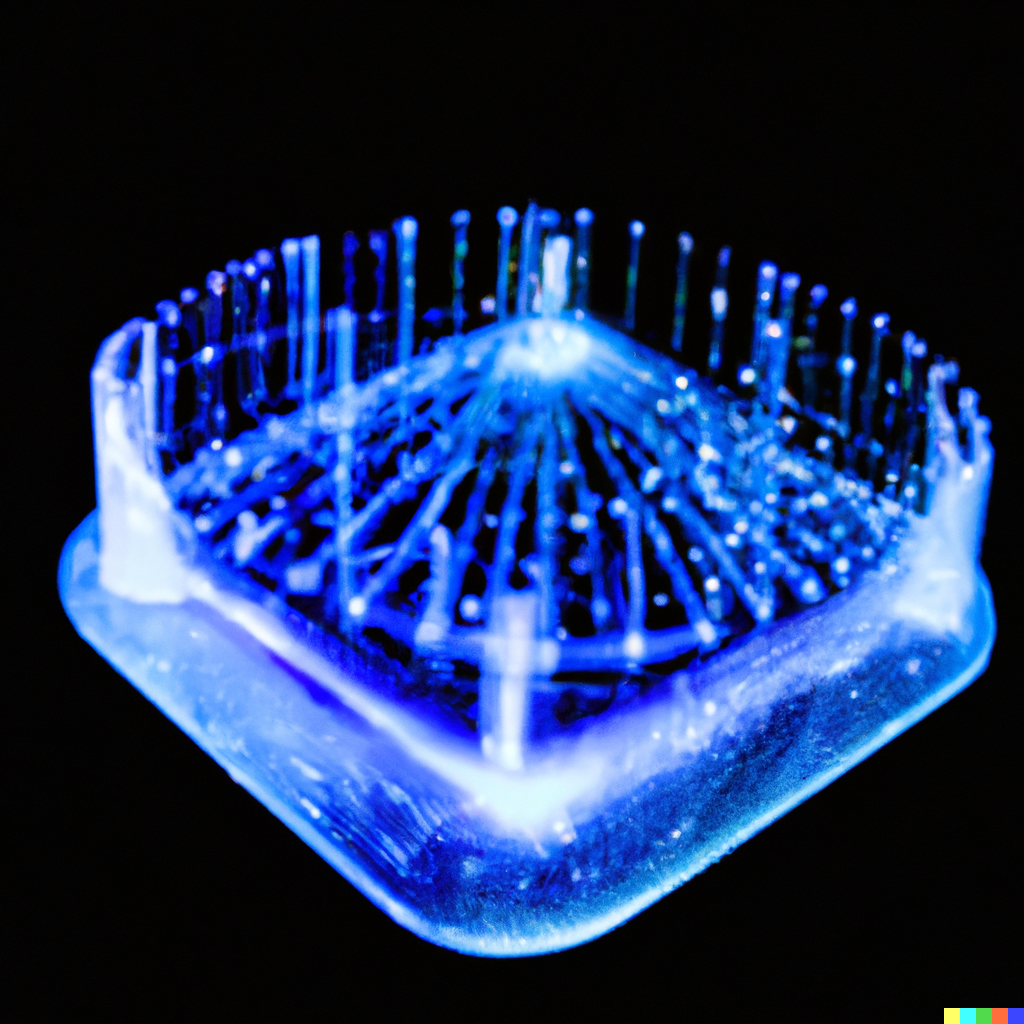
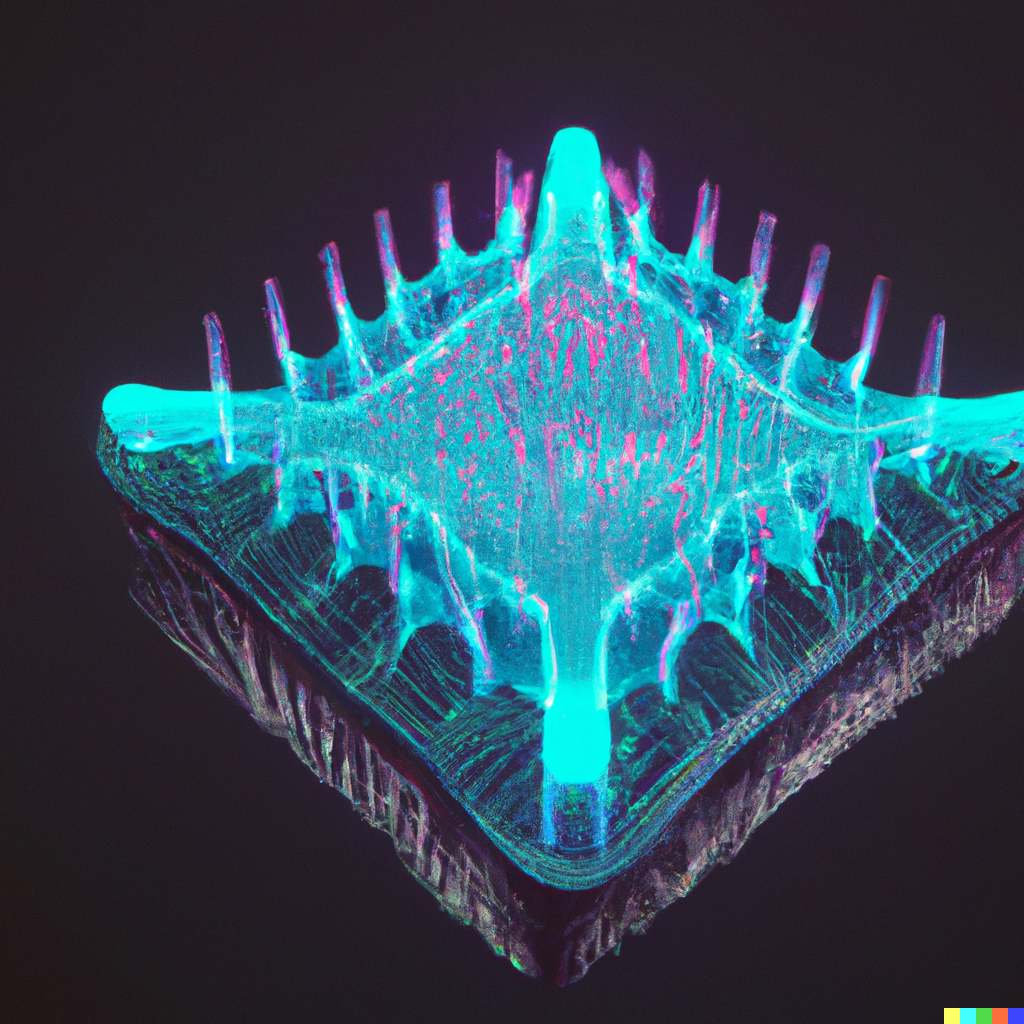
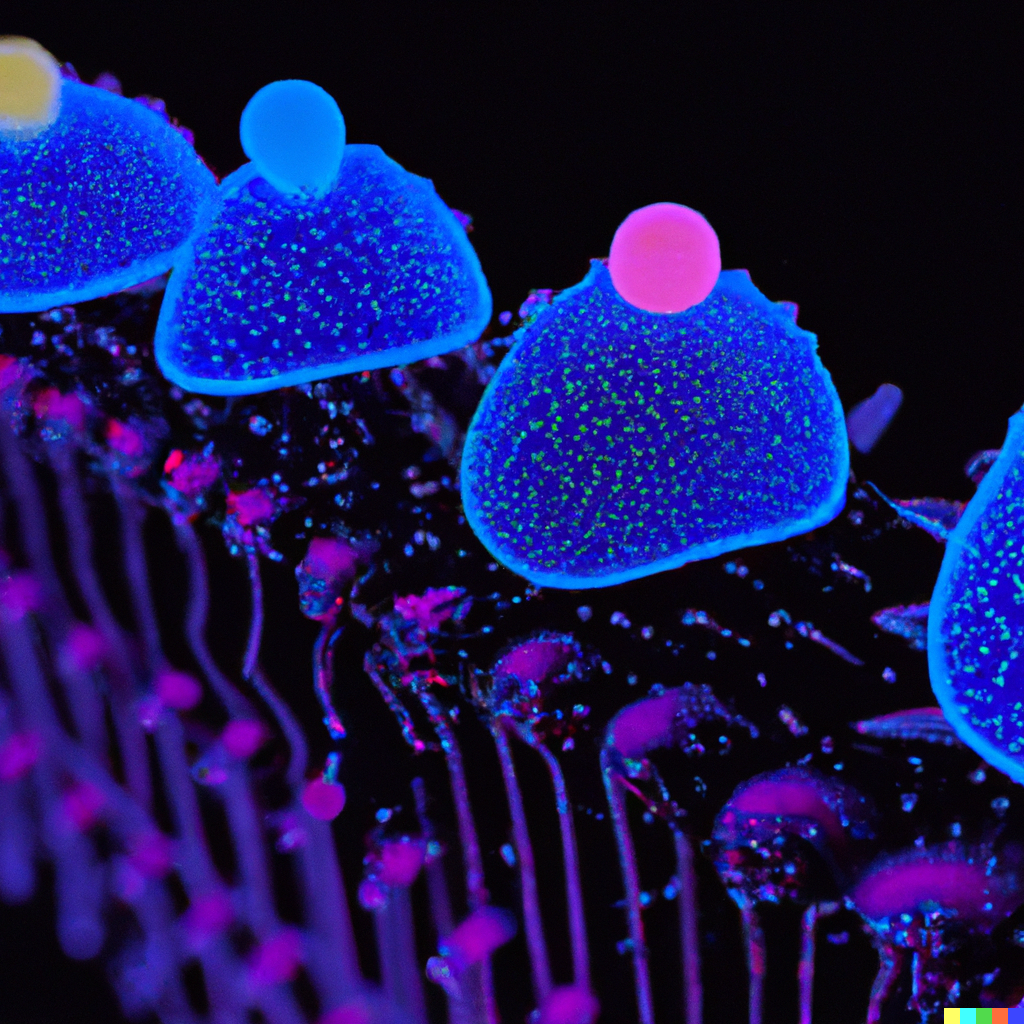
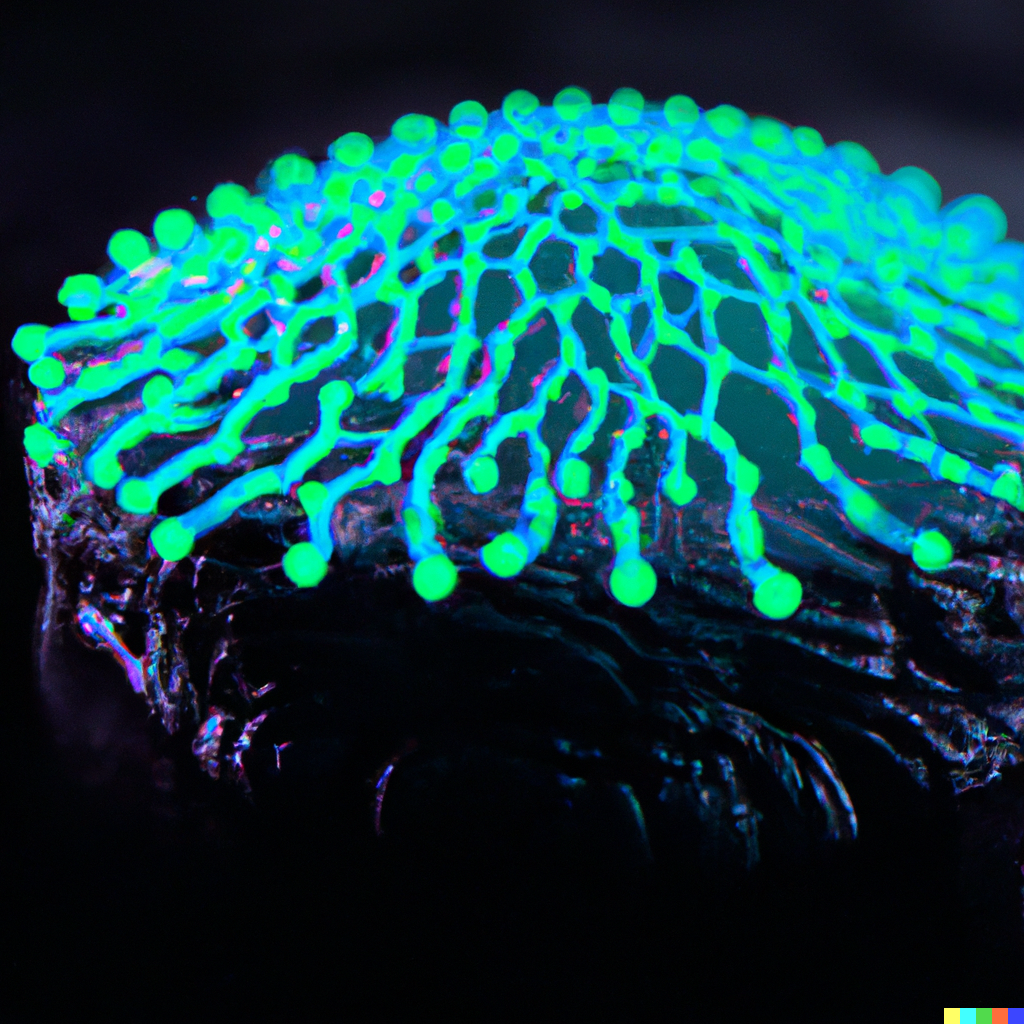
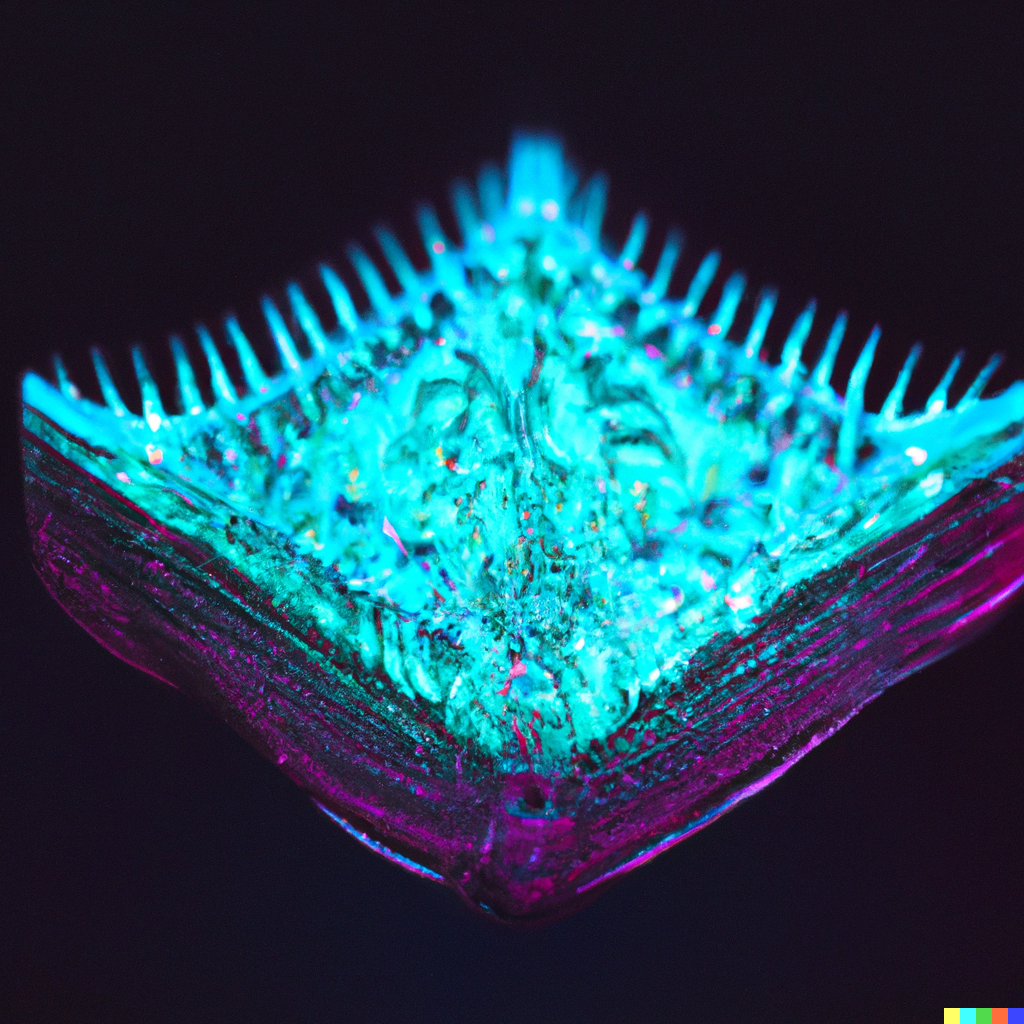
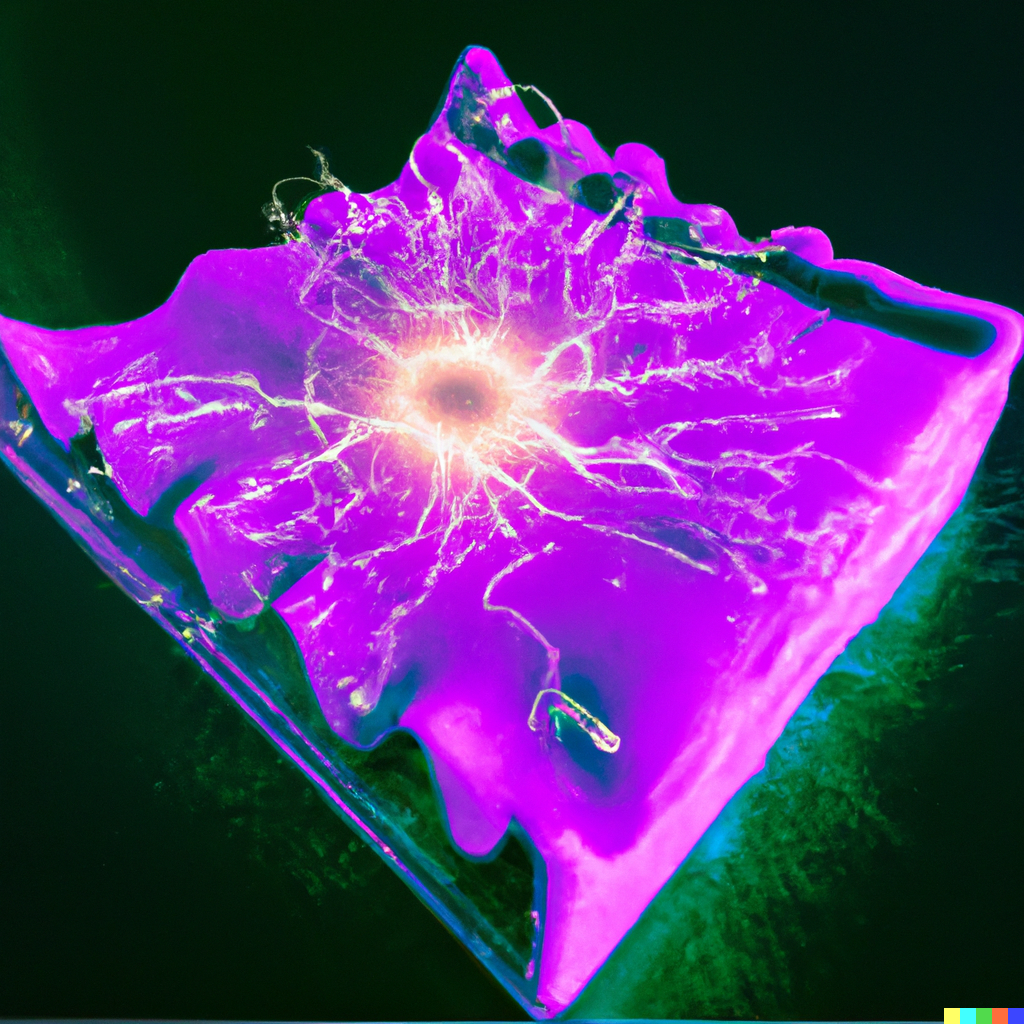
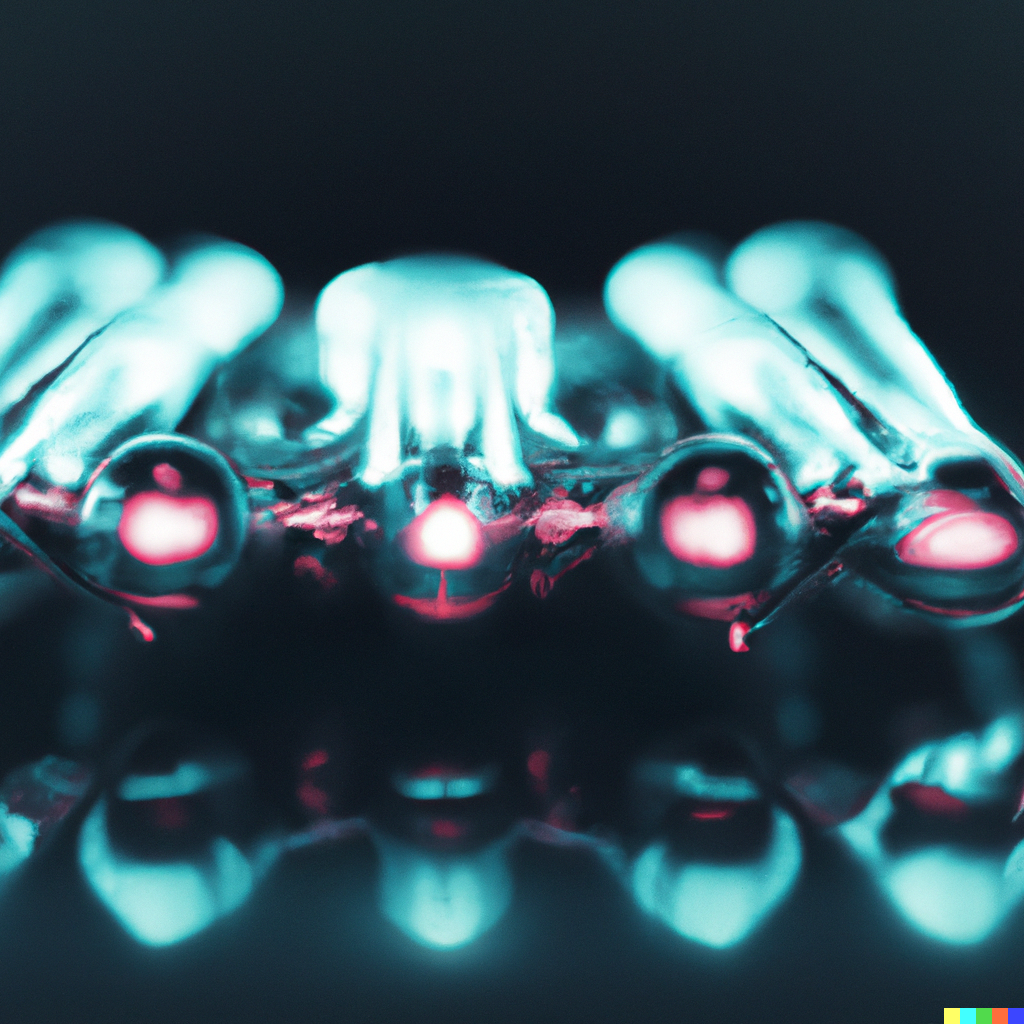
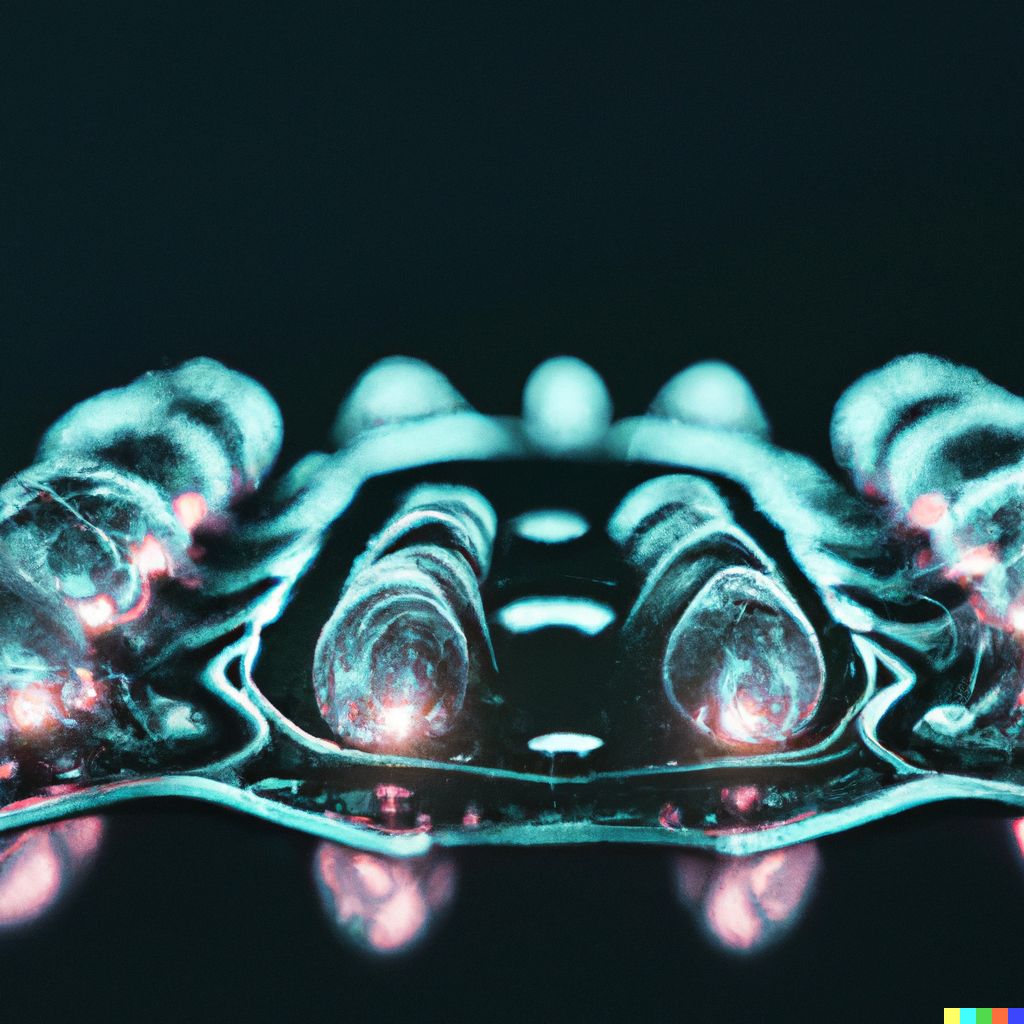
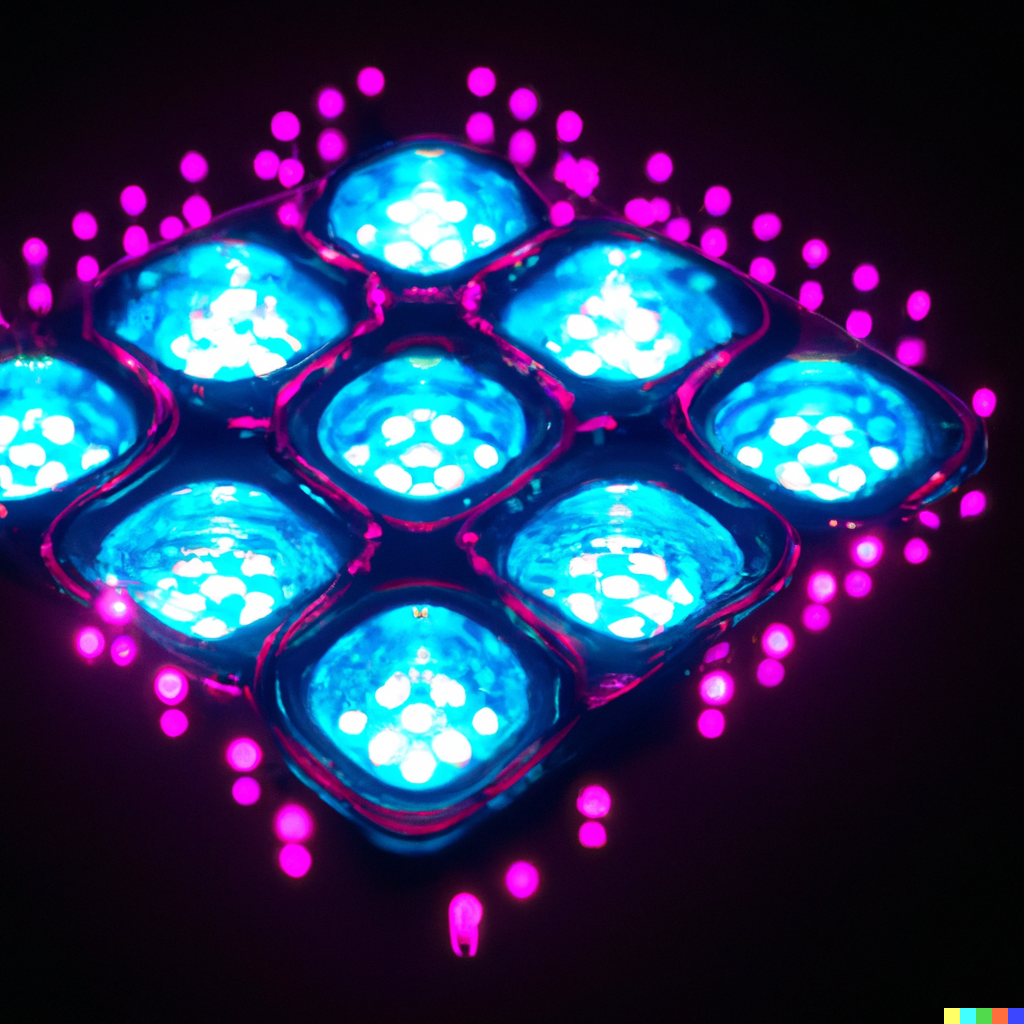
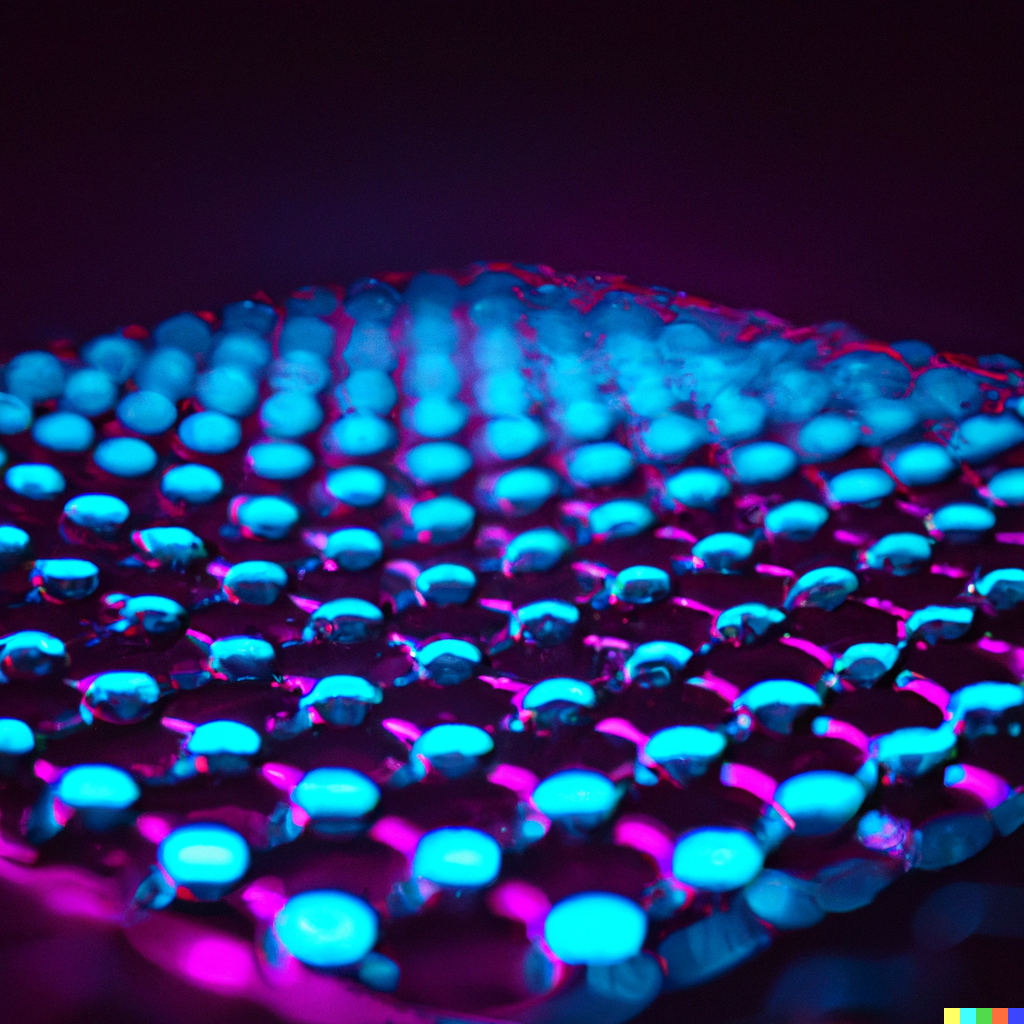
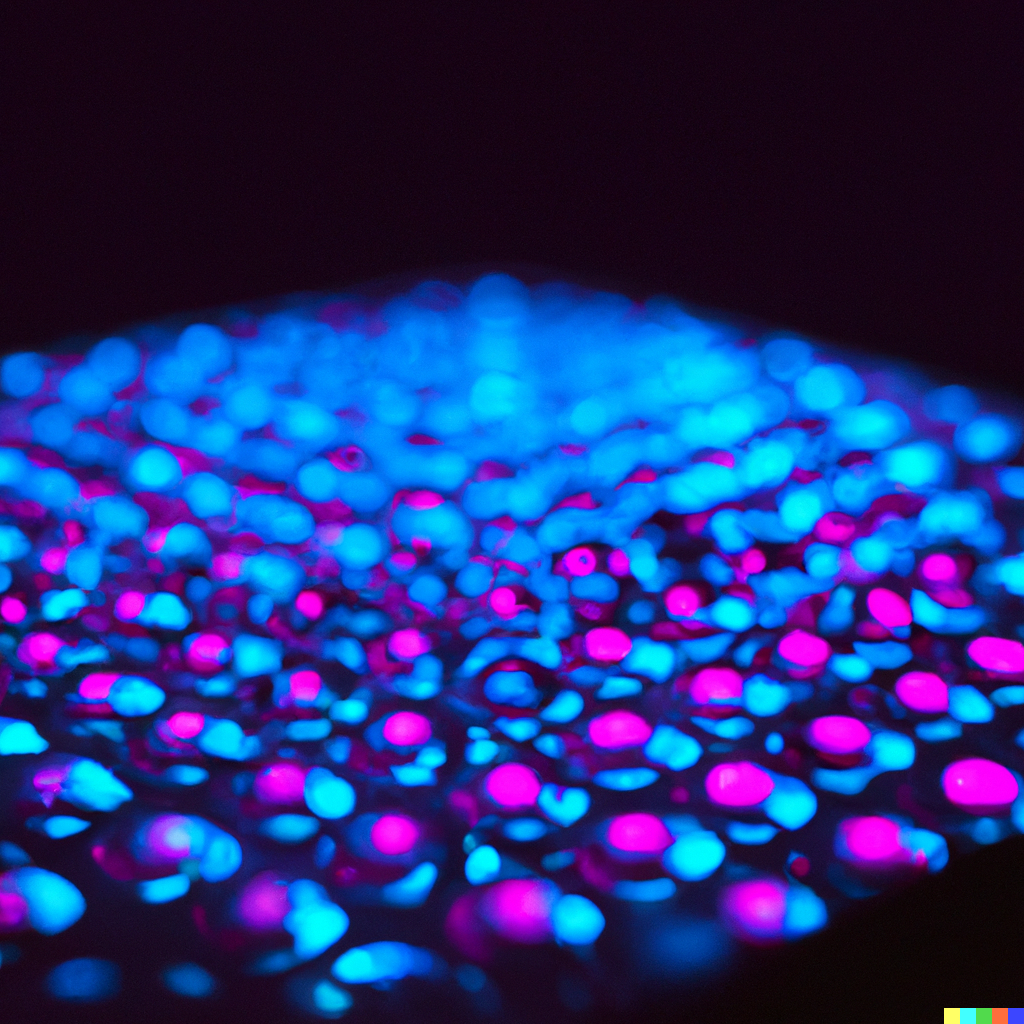
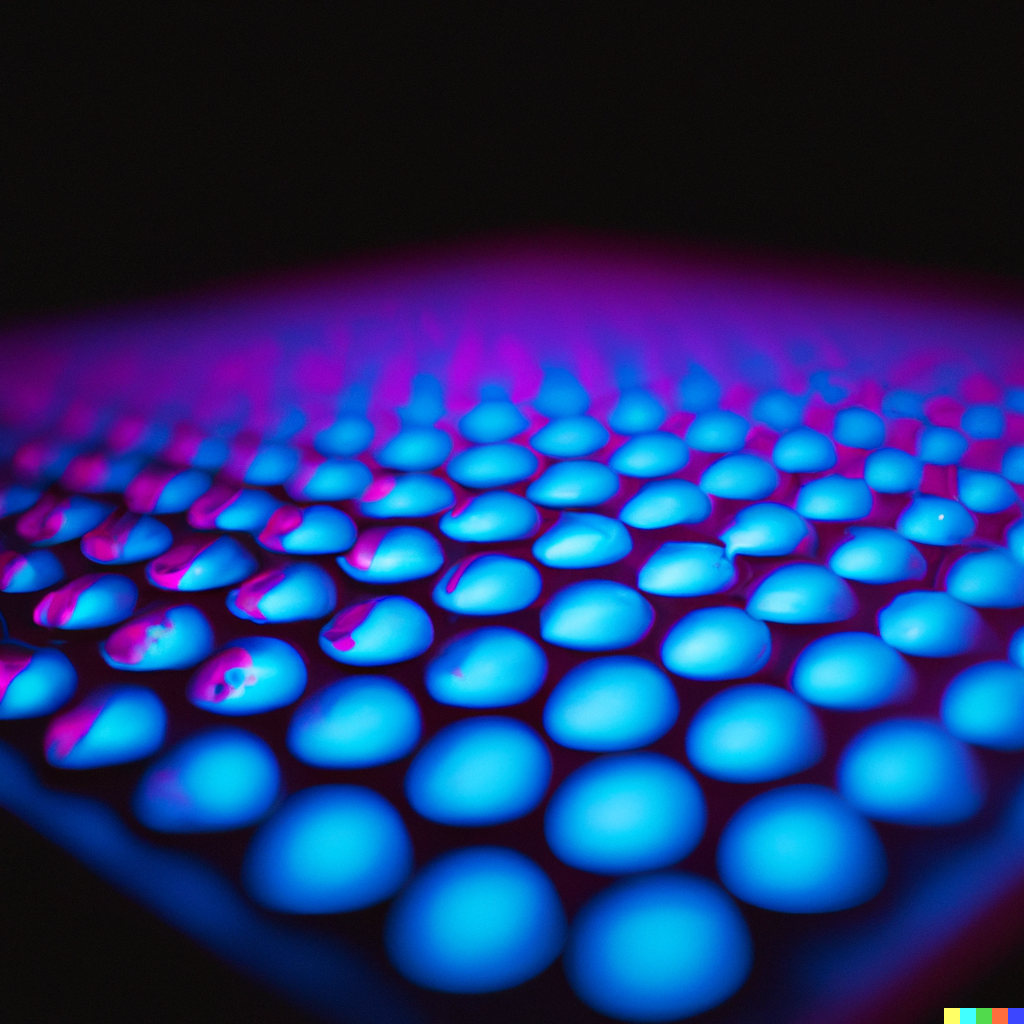
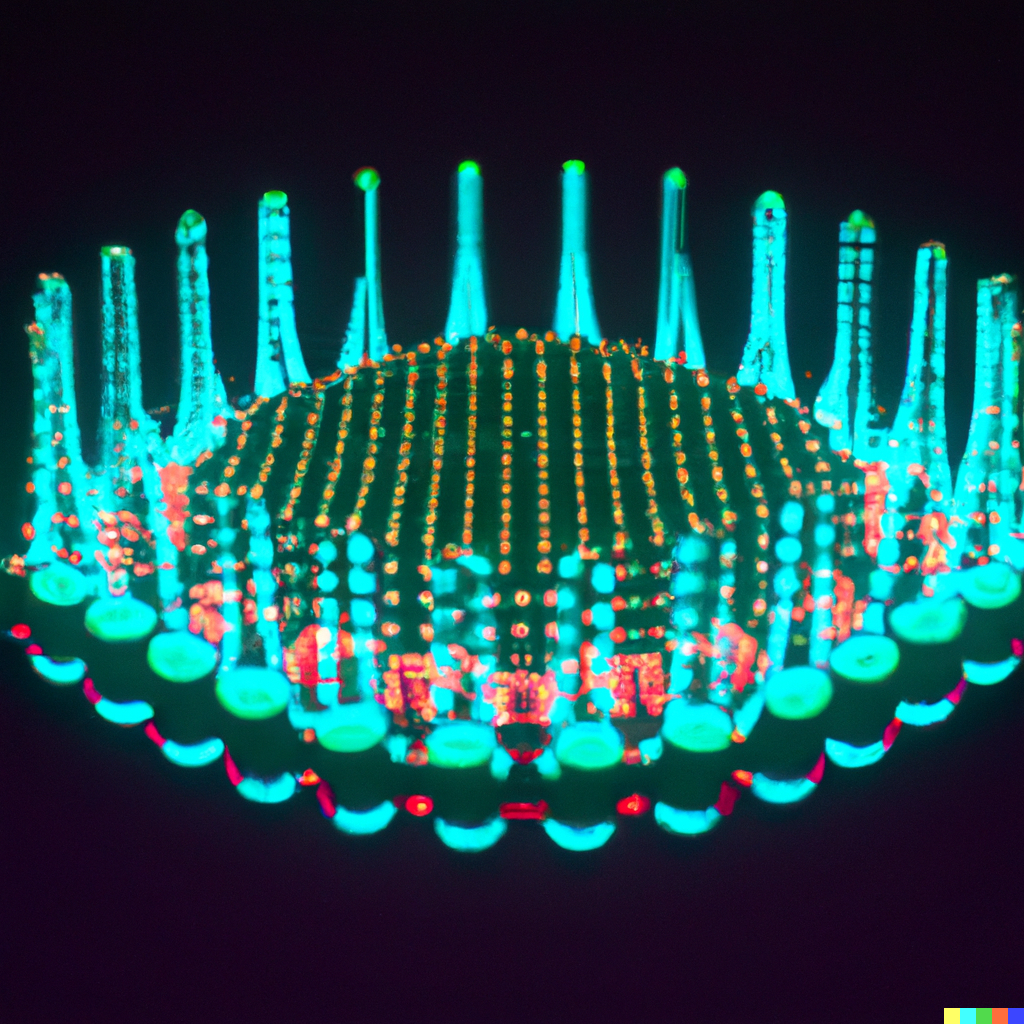
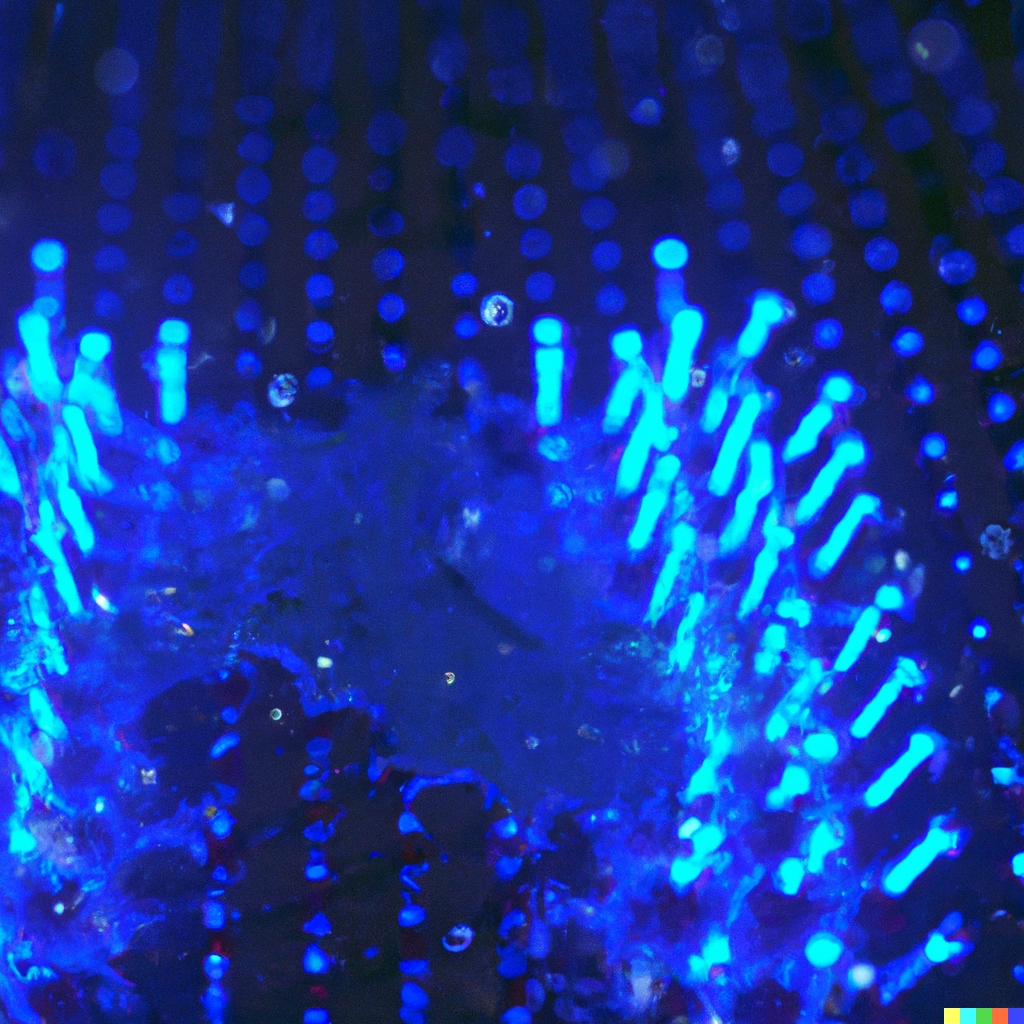
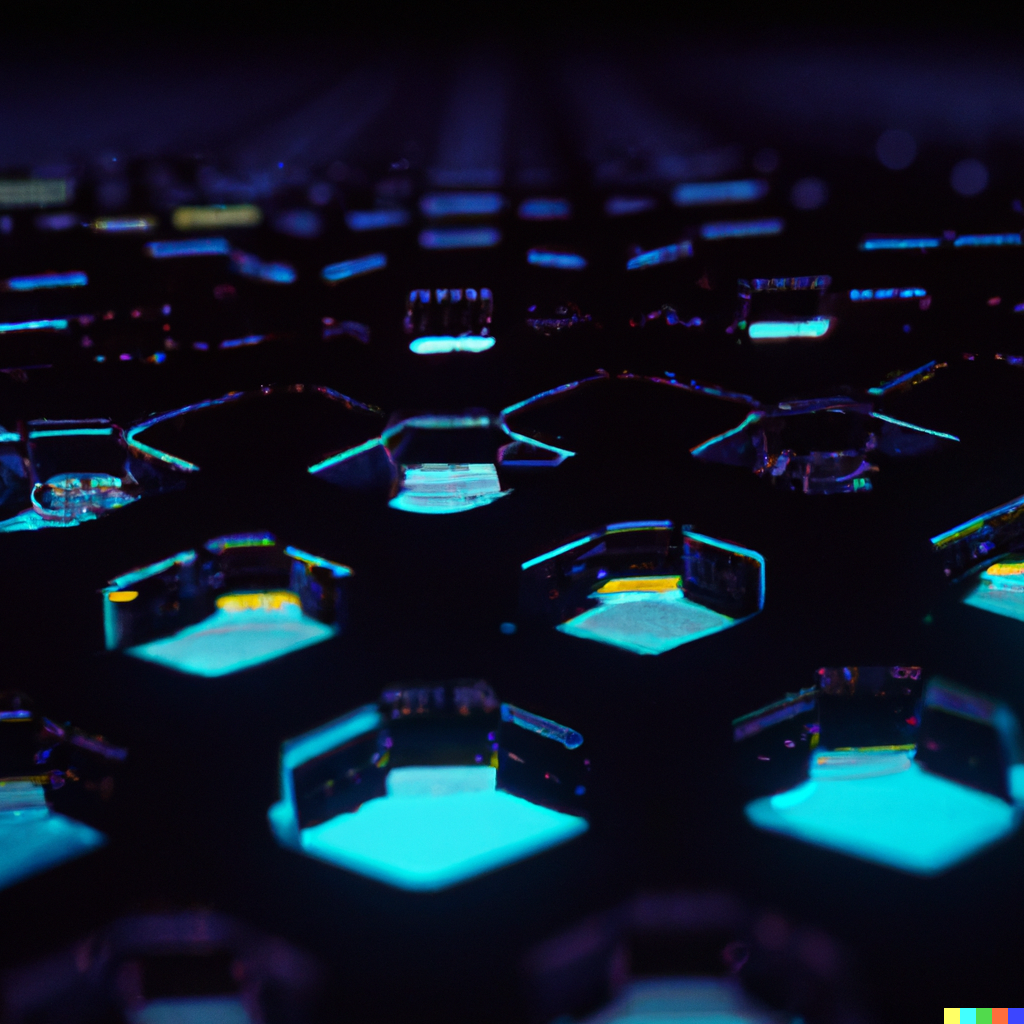
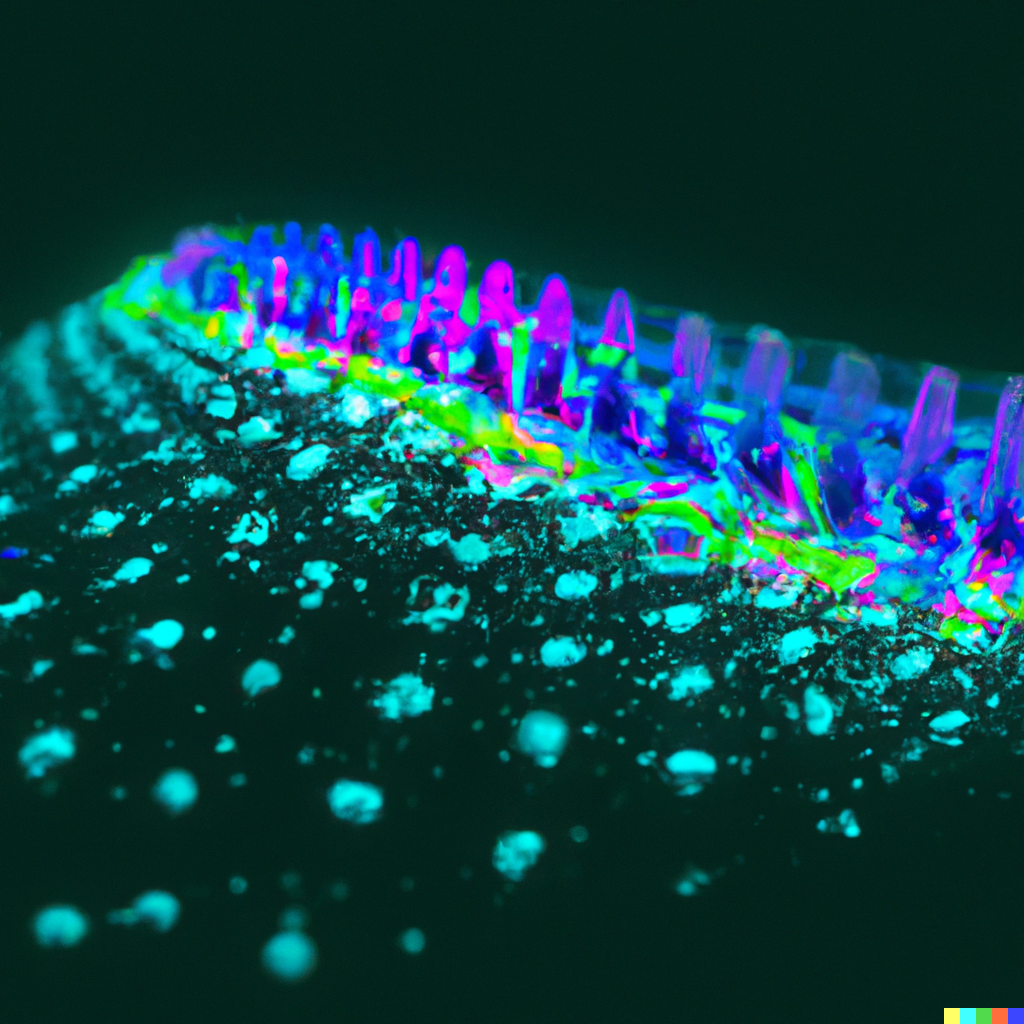
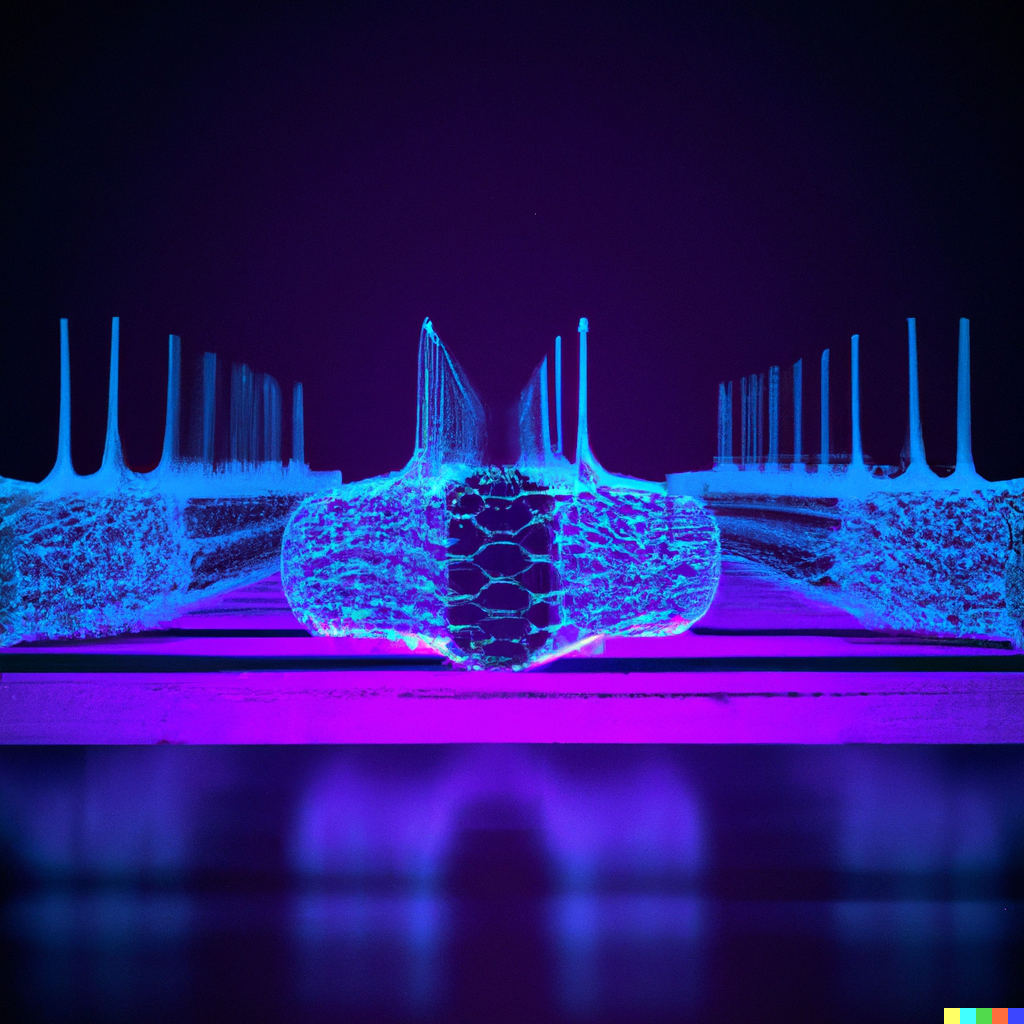
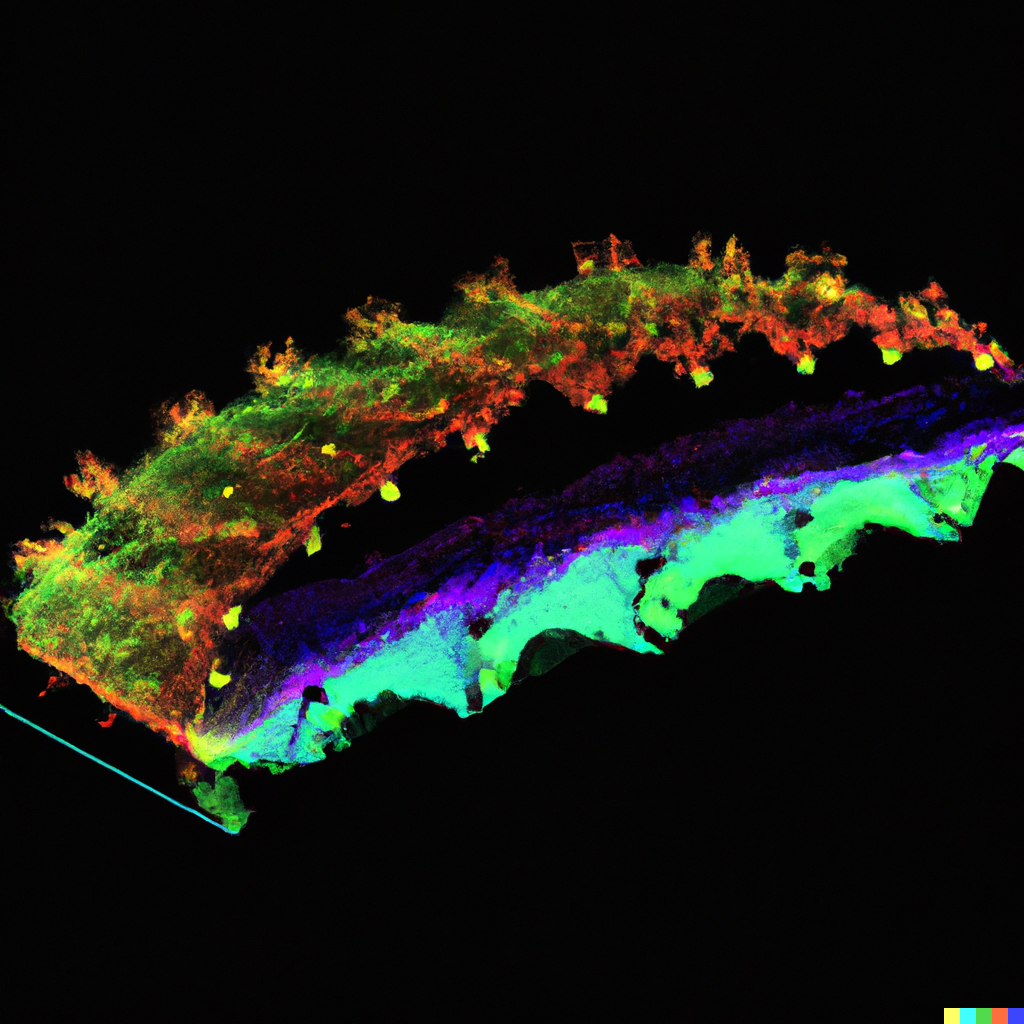
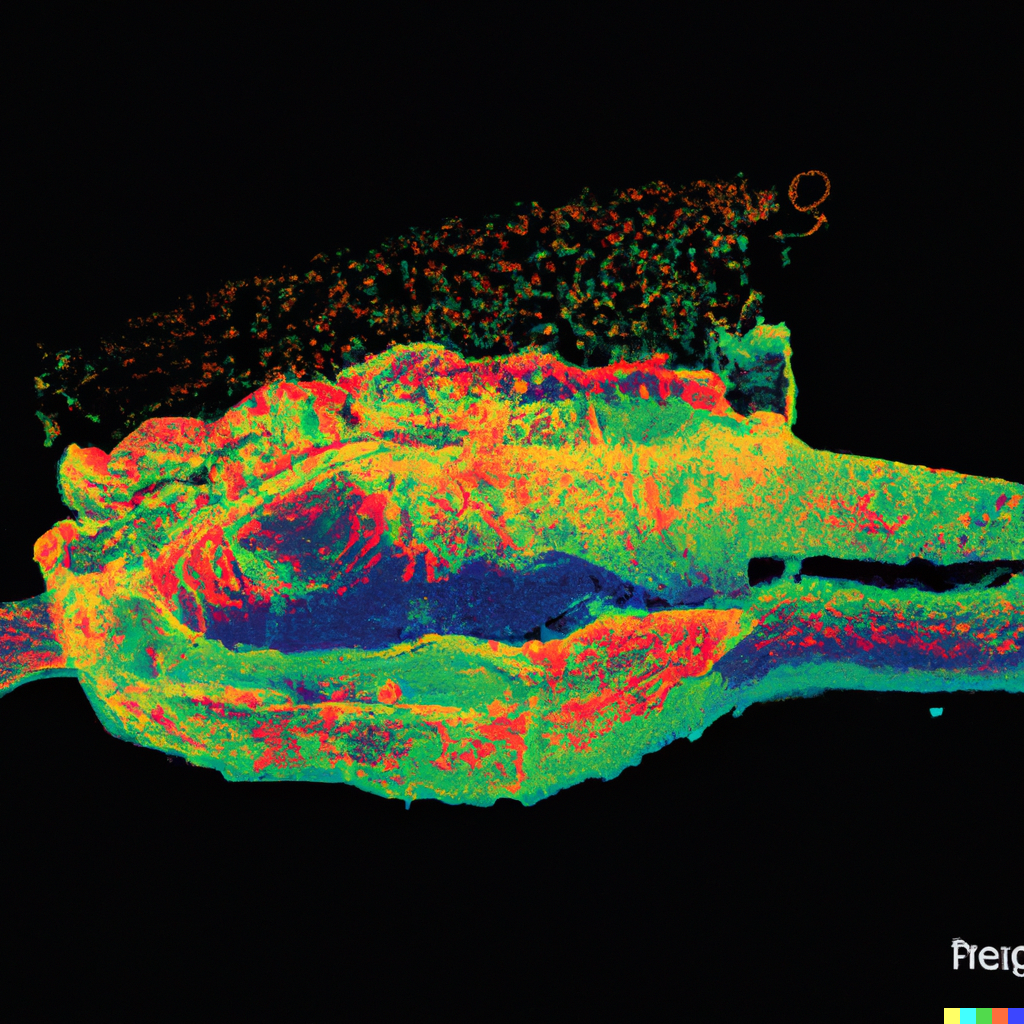
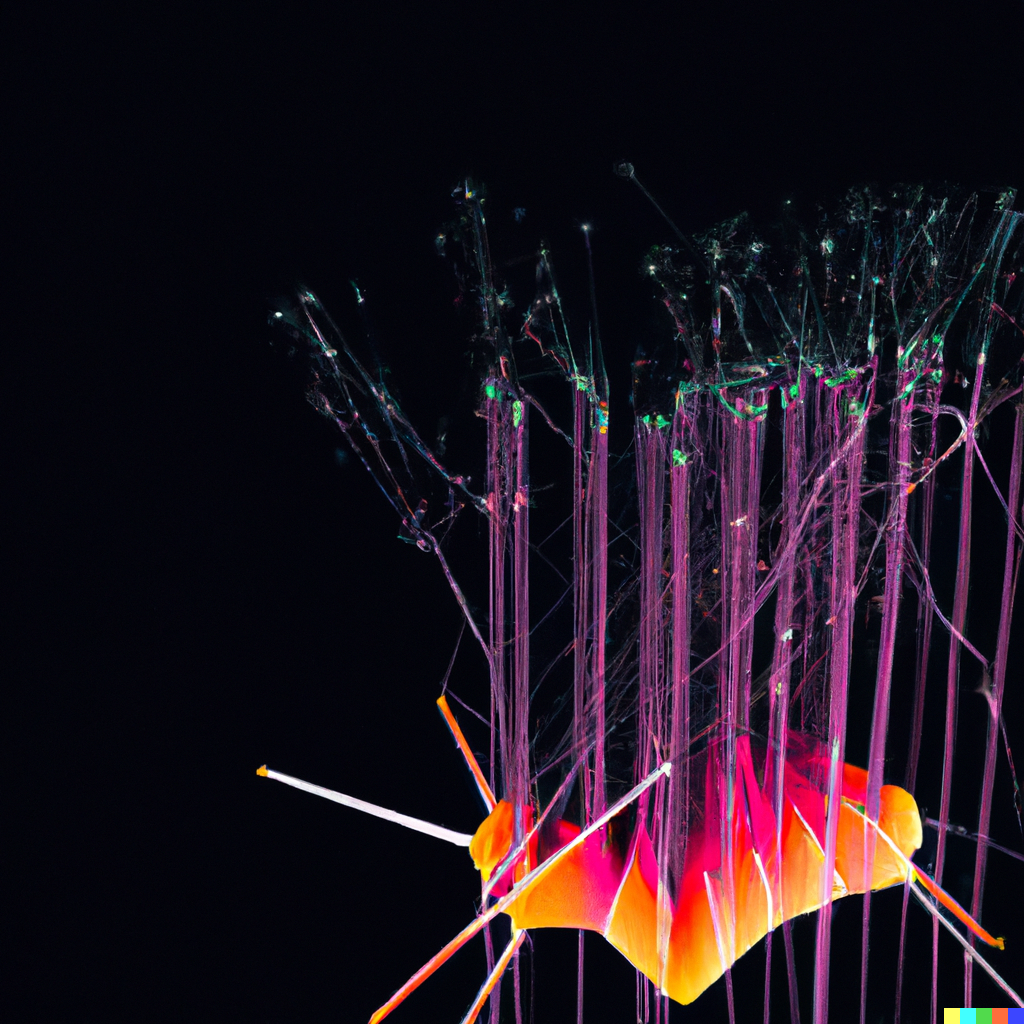
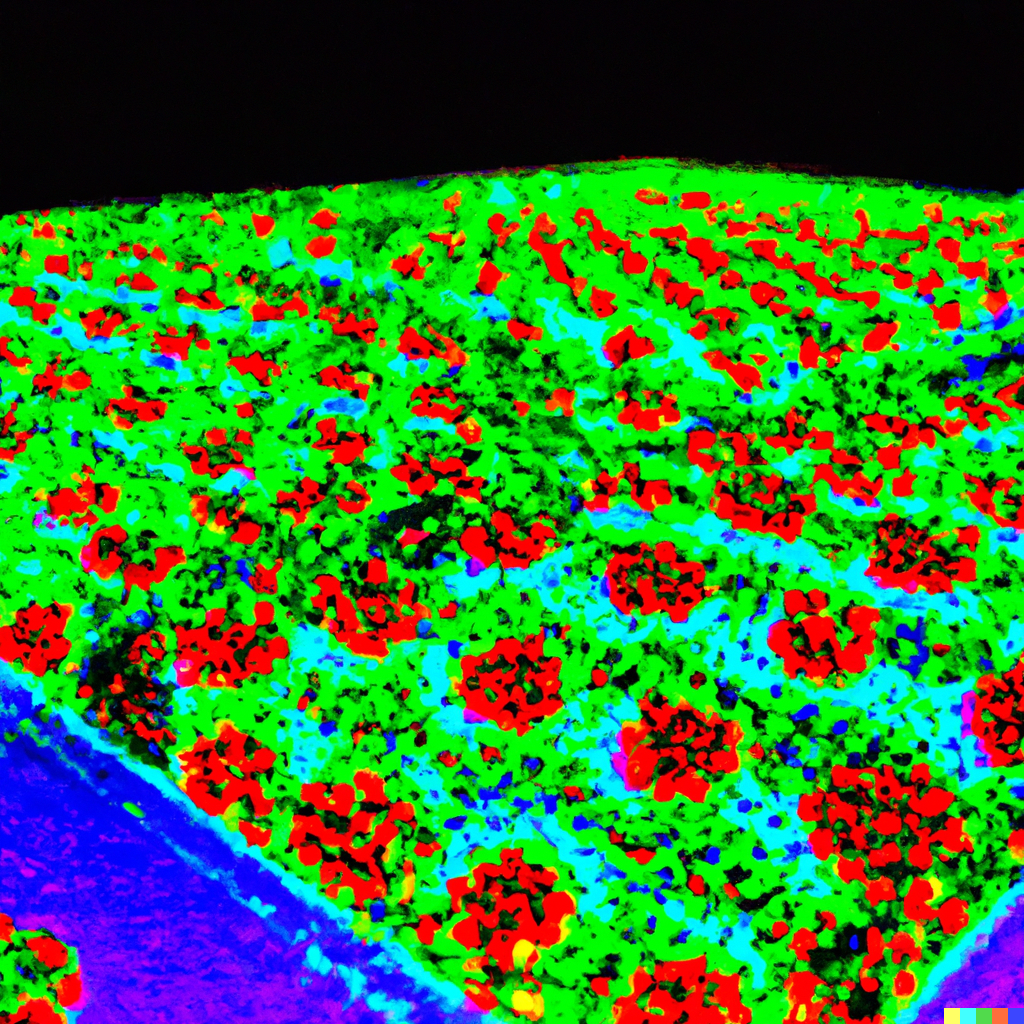
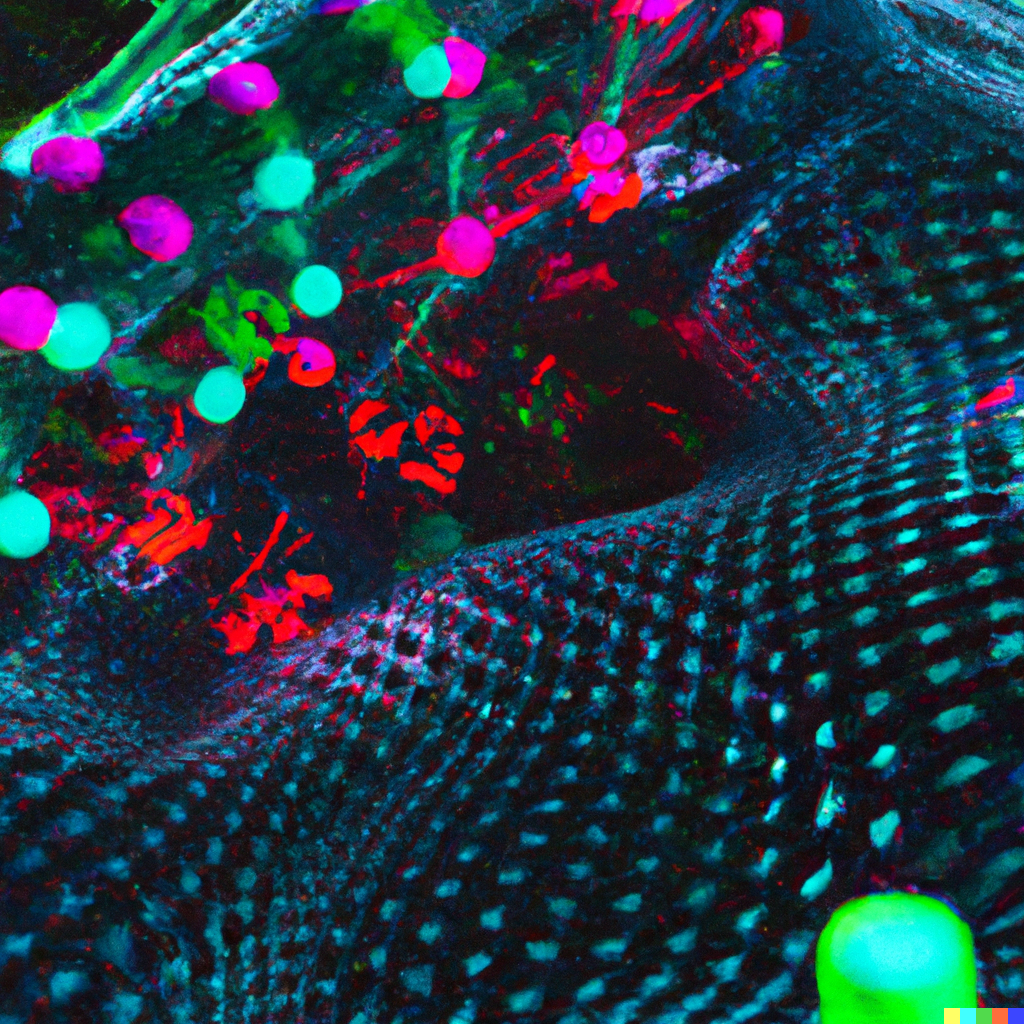
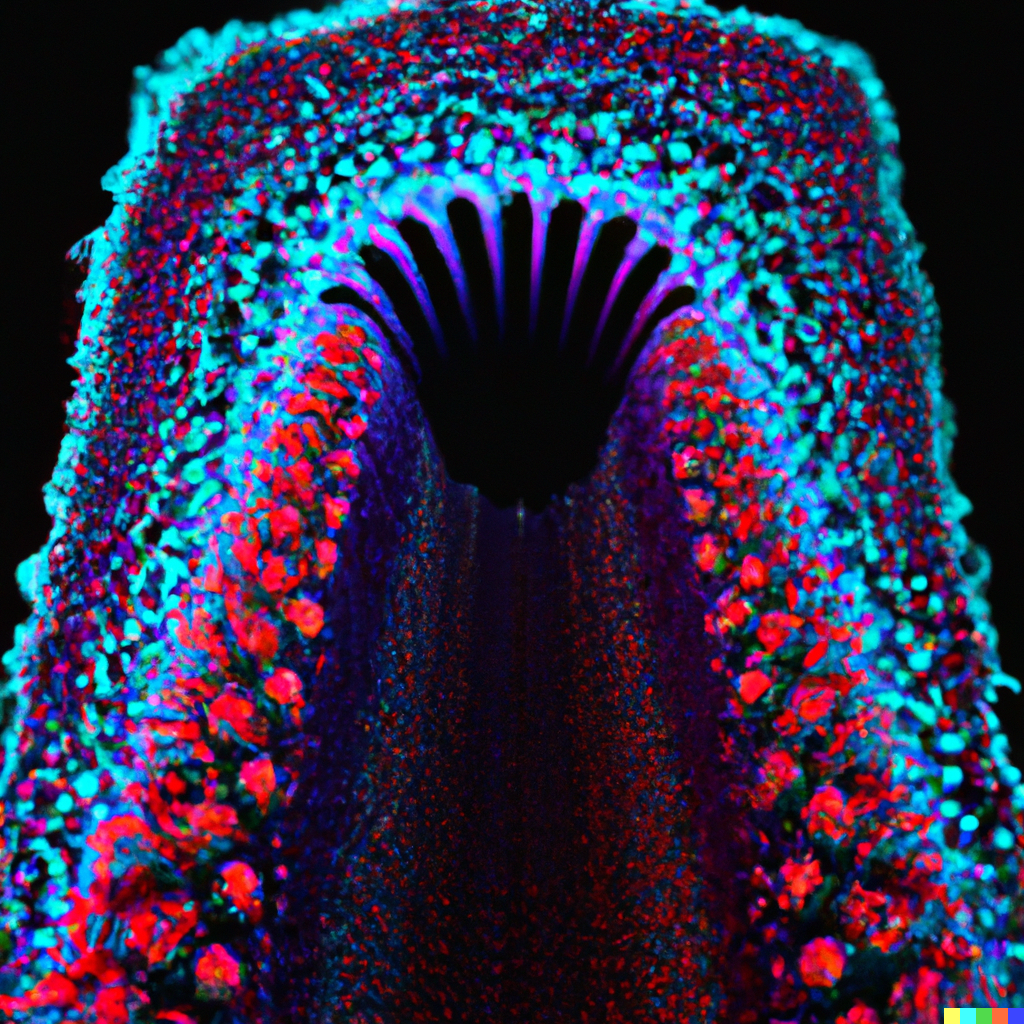
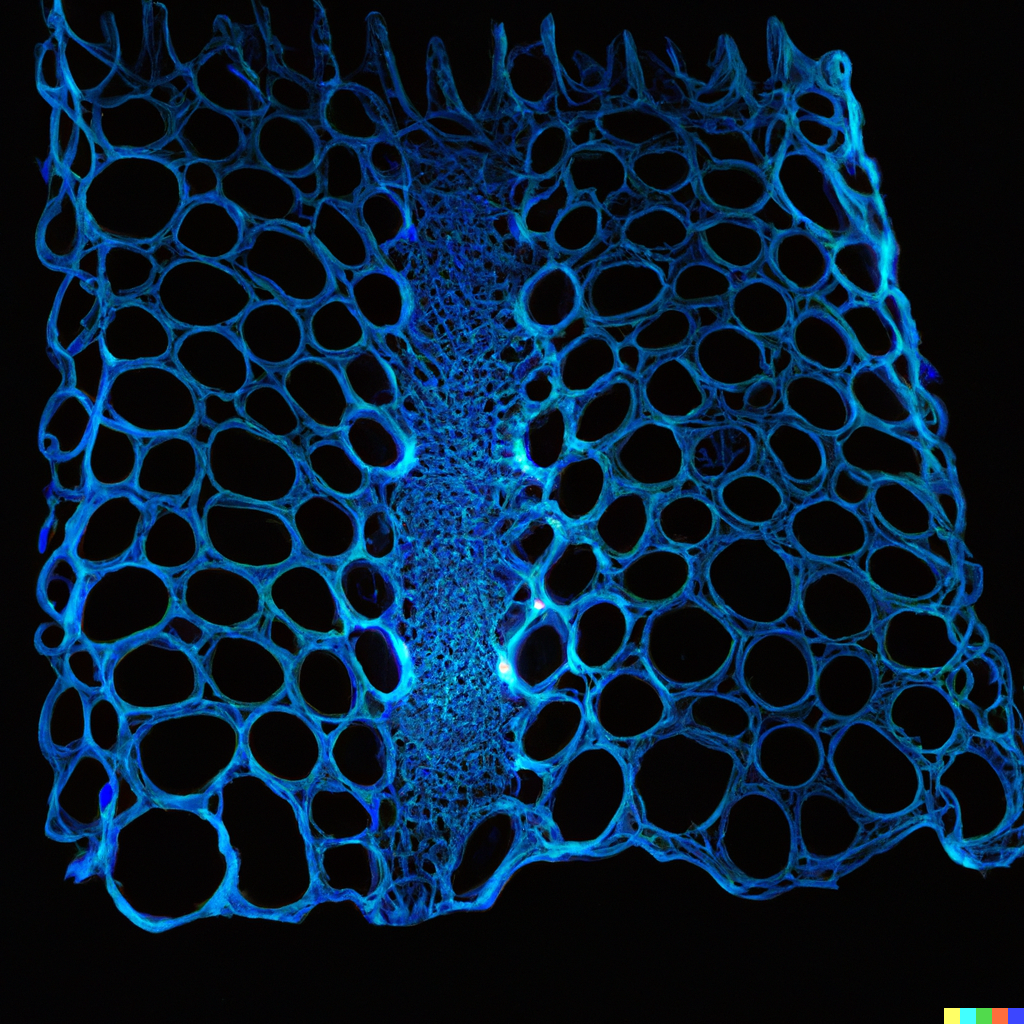
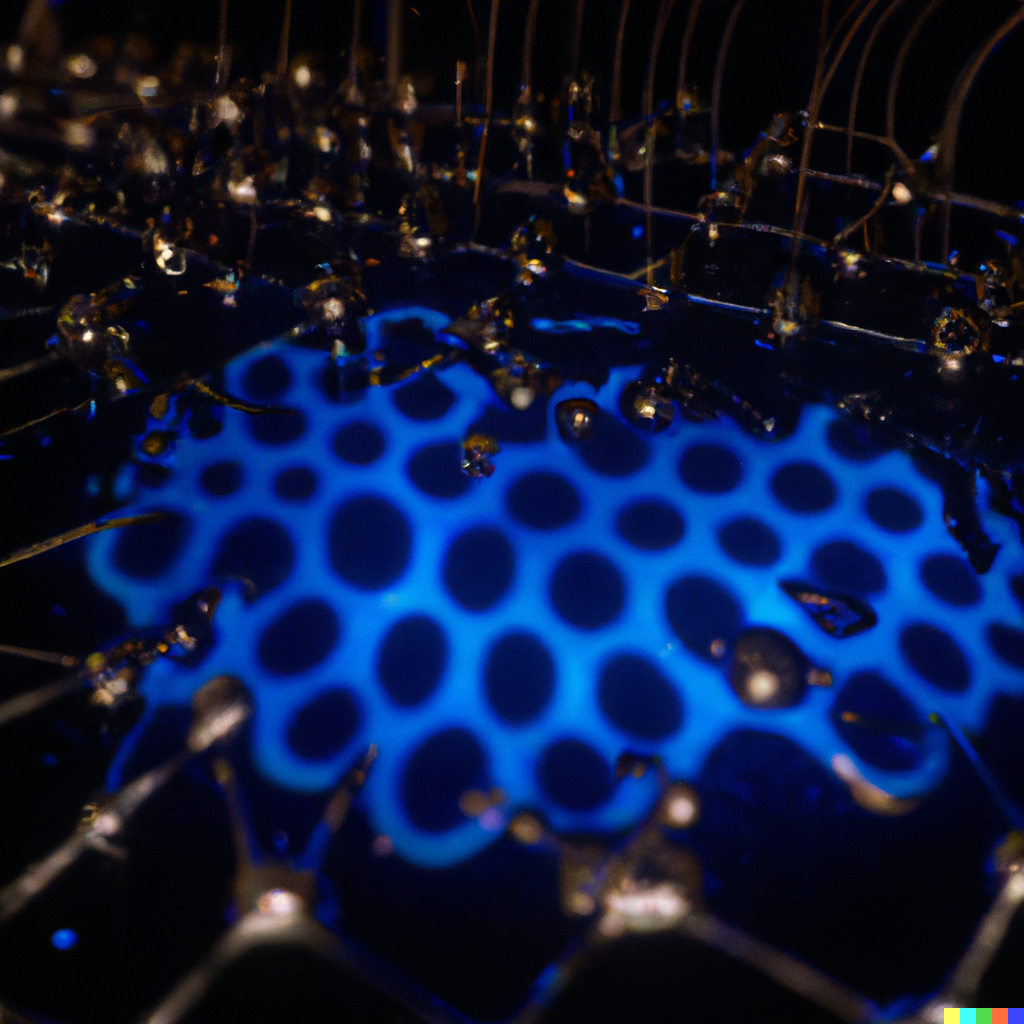
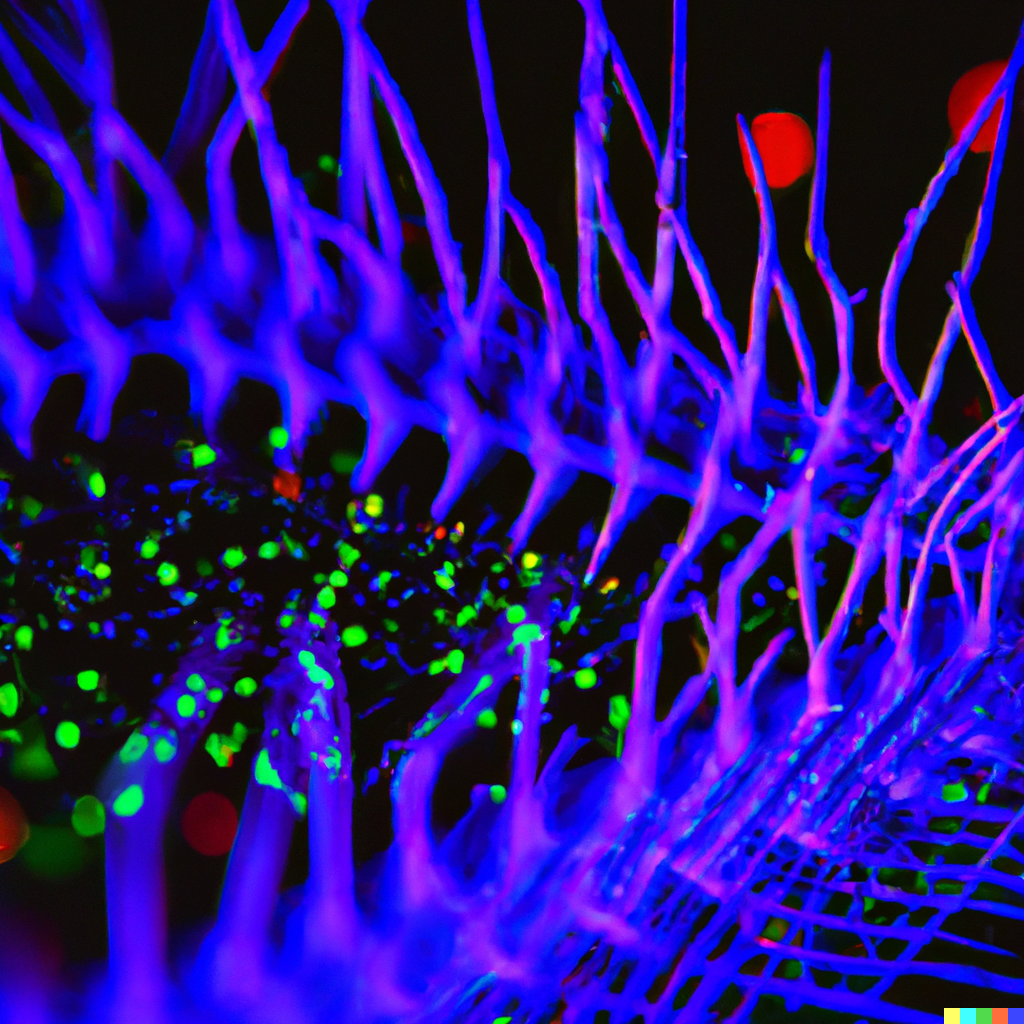
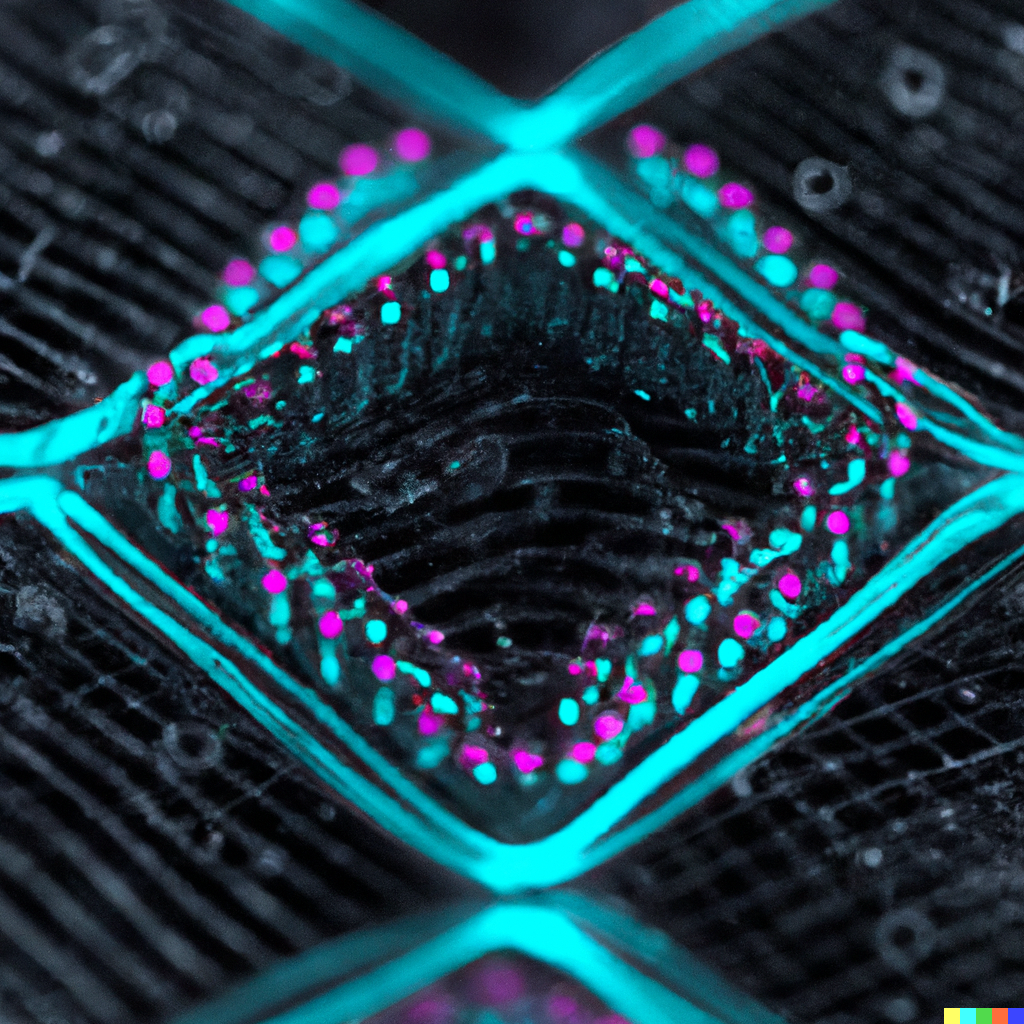
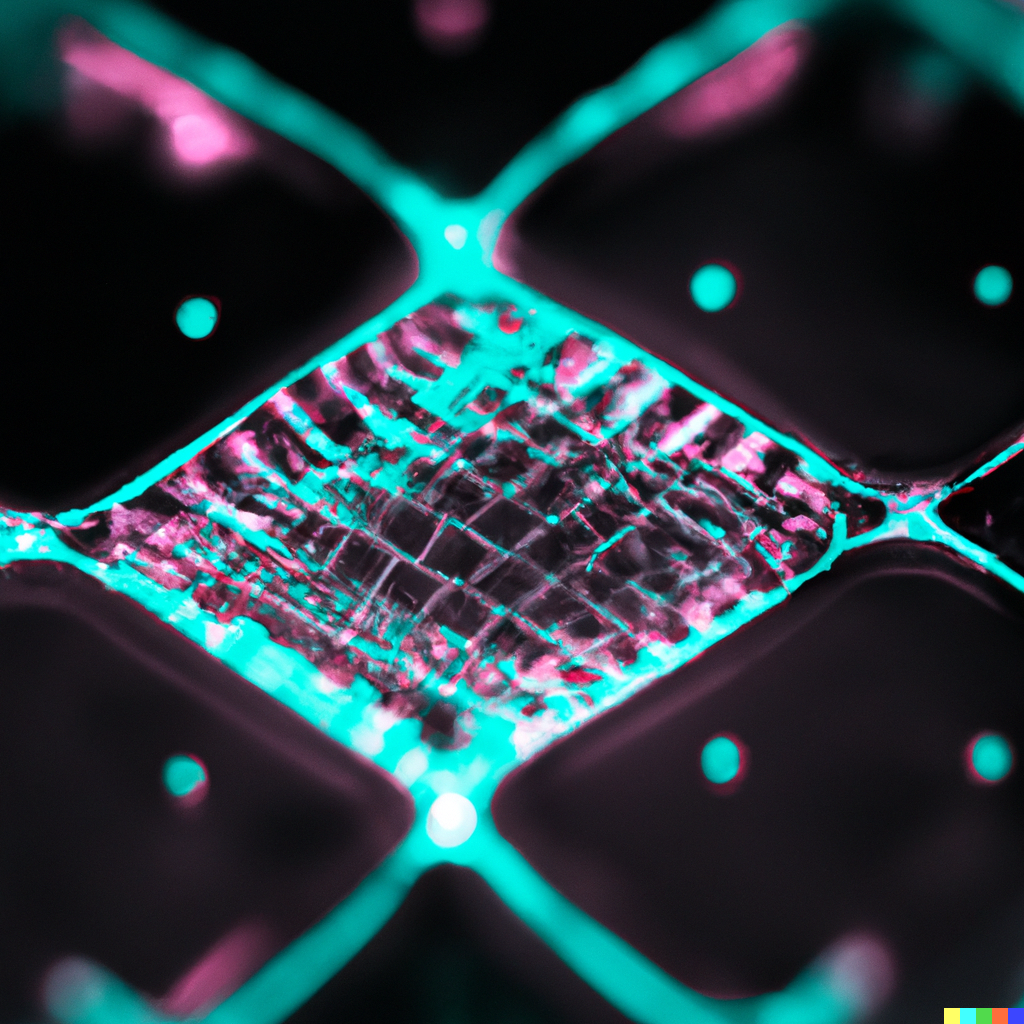
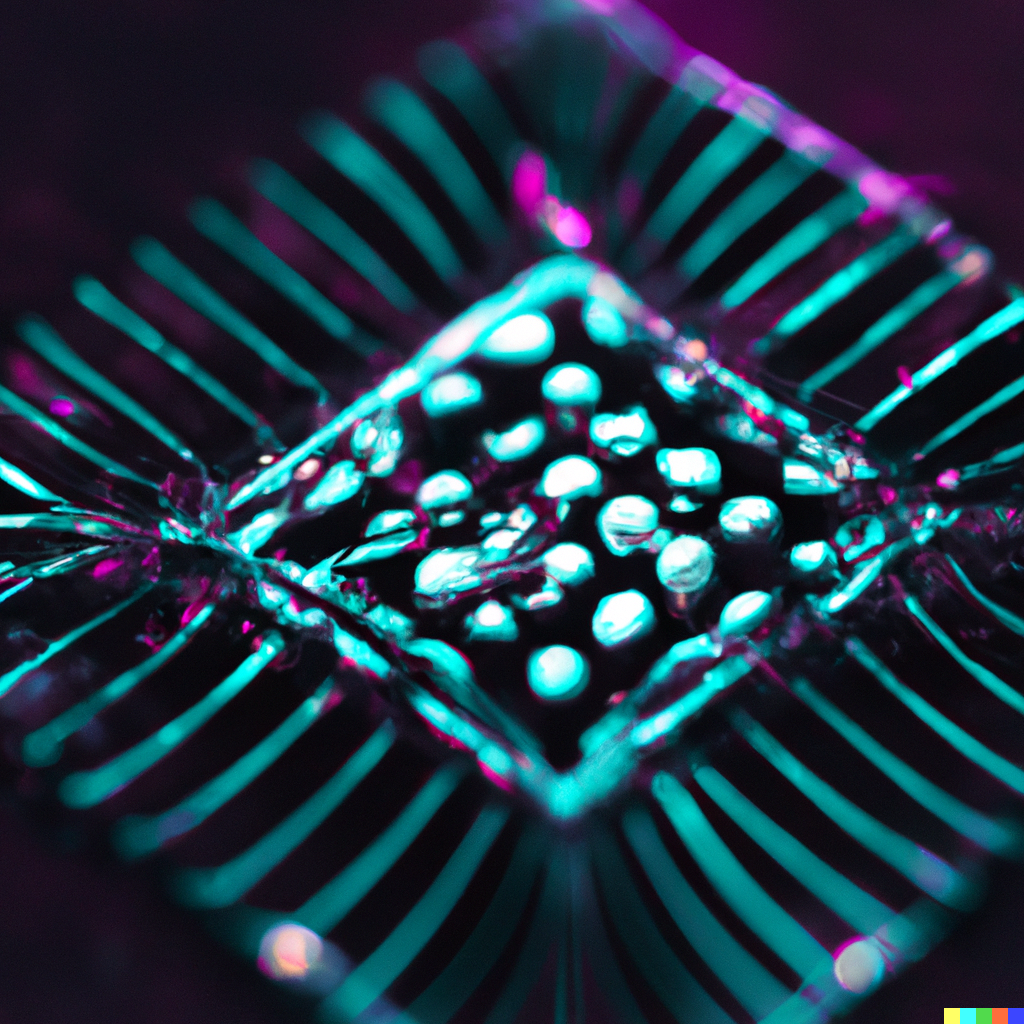
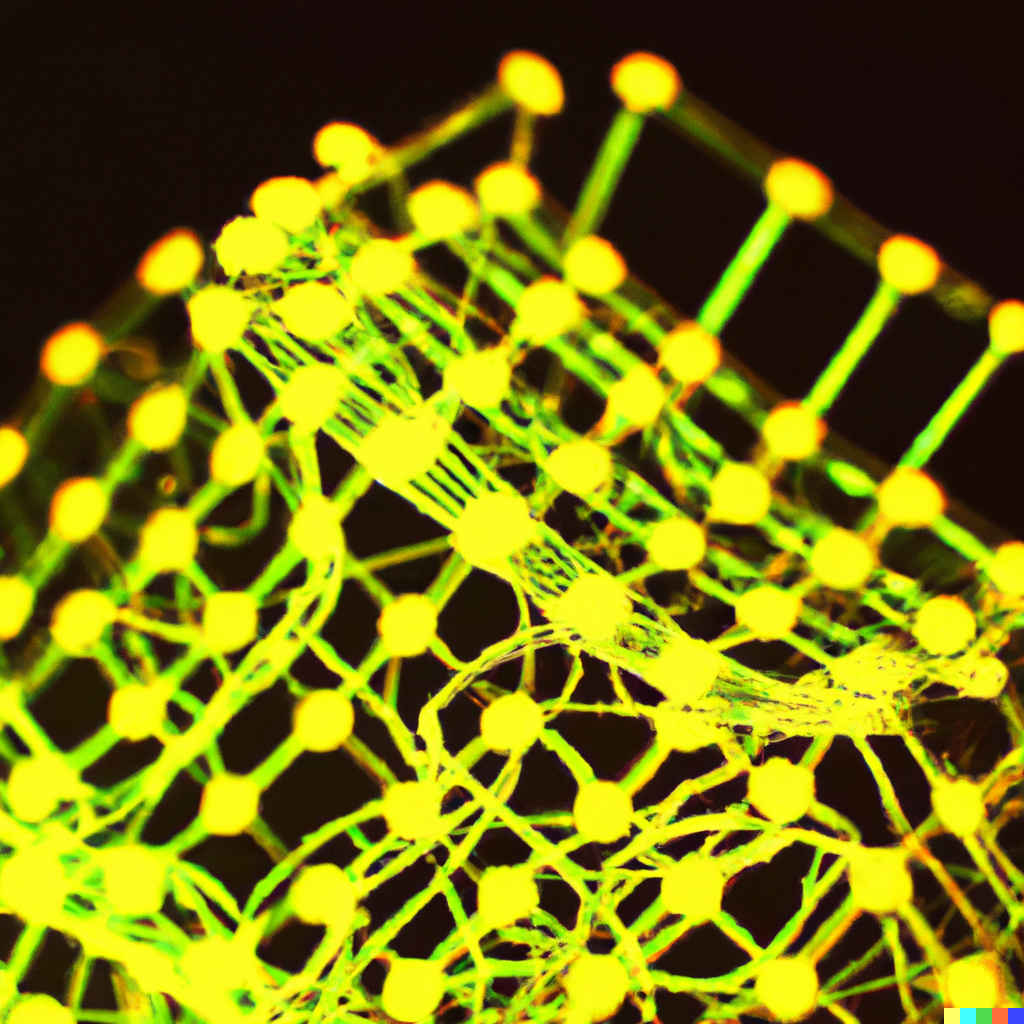
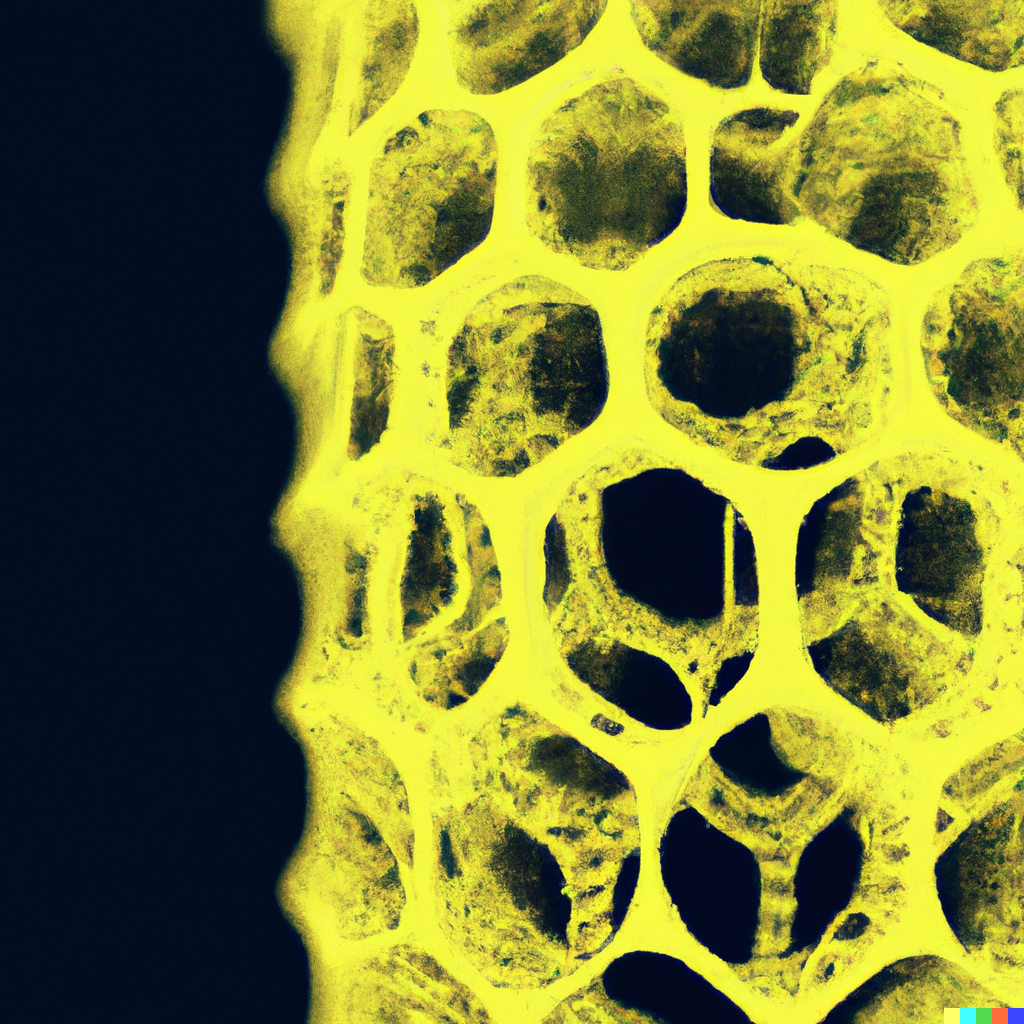
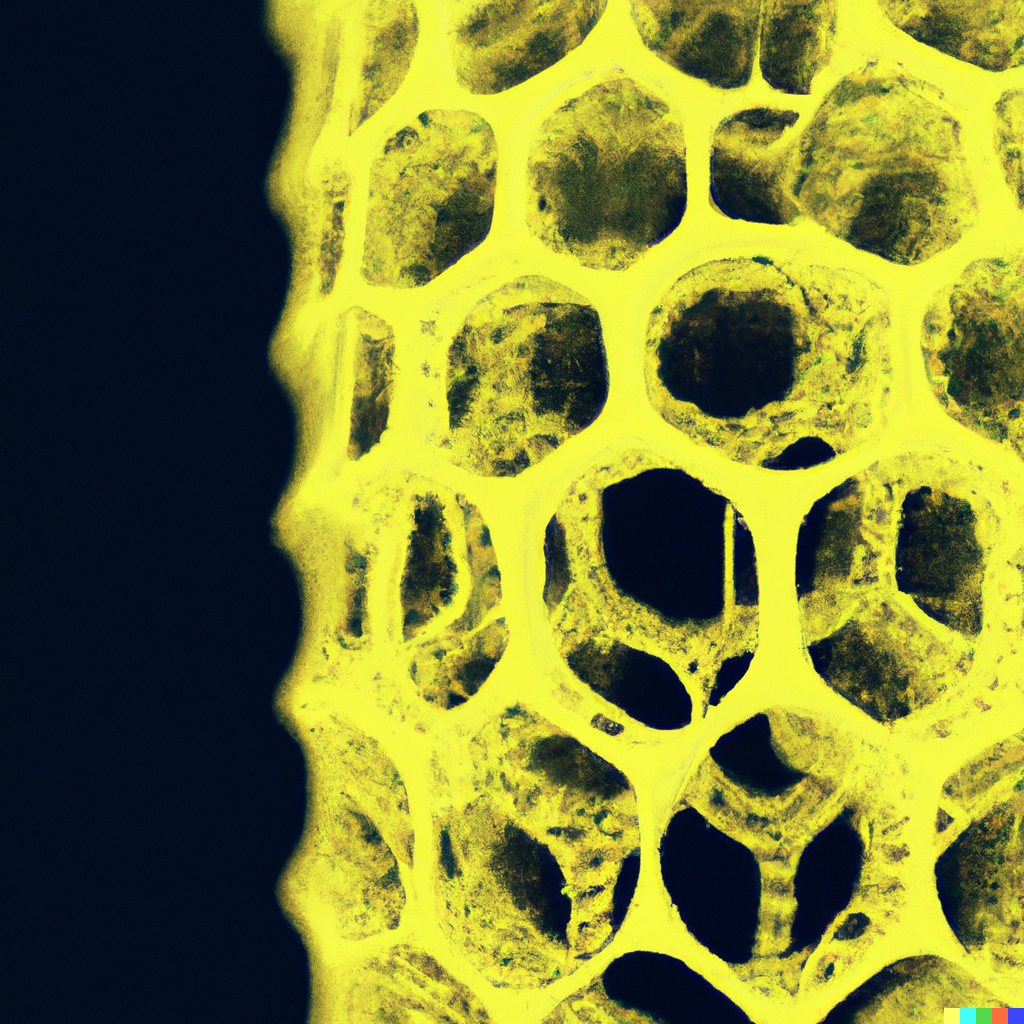